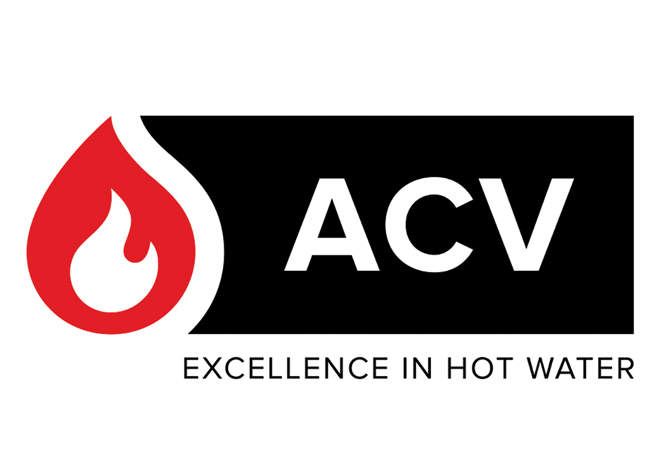
Hot water systems, which are employed in practically every occupied building in the UK, are increasingly important elements in the design of sustainable and resilient built environments. As the standard of thermal performance in the building envelope improves, the heat required for the DHW becomes a more significant proportion of a building’s heat demand. In smaller low-rise buildings, systems supplying hot-water outlets are increasingly being directly fed – without interim cold-water storage – so directly impacting the predicted demand on wholesome cold-water supply. Also, in larger buildings, advances in pumped, pressure controlled water systems are delivering increased precision in design flowrates. Alongside this, the increasingly urgent need to reduce the lifecycle carbon impact of engineered systems means that designers must account for both the embodied, as well as the operational, carbon impact.
Optimisation is therefore the key, but without appropriate data on hot-water consumption to inform that system design, it is very unlikely that systems will be installed and operate economically – in water usage, cost, and carbon terms – while also being able to meet the primary need of delivering hot water to building users.
In Mohammed’s recent paper1 it is noted that water use in buildings has reduced significantly in recent years, owing to increased user awareness of environmental impacts and the adoption of water efficiency measures. However, the paper reports, despite considerable changes in water end use and users’ behaviour, there have been no corresponding changes in sizing methodology and no significant update to the design equations. Therefore, as reported by Tindall,2 it would appear this leads to oversizing of water systems in buildings.
The method adopted for estimating the demand on the hot-water system will depend on the type and application of the system that is being designed, and will also be subject to the building layout, distribution and type of water outlets, and the pattern of hot-water usage across the day. CIBSE Guide G3 relates that the size of plant required is determined by the draw-off rate and pattern of consumption in each day, and that the hot-water storage capacity should be related to both the design consumption and recovery rate. In the UK, hot-water production and storage temperatures must comply with Health and Safety Executive (HSE) requirements4 in order to minimise the growth of legionella bacteria. This requires that, if there is hot-water storage, it should be stored at least at 60°C and distributed so that it reaches a temperature of 50°C (55°C in healthcare premises) within one minute at the outlets. In anything larger than small commercial systems, this would likely require DHW recirculation to maintain the minimum required temperatures at the outlets.
UK hot-water systems will normally be arranged as one of three main types:
Storage system – this is often considered as a traditional system in the UK. In both CIBSE3 and Chartered Institute of Plumbing and Heating Engineering (CIPHE) guidance,5 the size of the store is established based on expected consumption over a particular time period that can be heated to the normal operational temperature from cold (10°C) in, typically, two hours.
Heat is generally provided from a gas- or oil-fired boiler, using a primary circuit to transfer low temperature hot water (circa 80°C flow) through a heat exchanger within the hot-water store. Such systems normally operate with a daily or weekly cycle of heating up, DHW consumption, and then partial cool down before recommencing the heating as the next cycle begins.
The store relies on maintaining a thermocline so that the hot water is drawn from the top while the cooler makeup water (or returning recirculating DHW) enters at the bottom of the store. The store can be topped up with heat at any time, and can provide good opportunities to aggregate output from various heat sources such as relatively low-output boilers, heat pumps, solar thermal panels and recovered heat.
Storage systems can provide an excellent response to unexpected peak demands (so long as they occur when the bulk of water is heated) but come with the disadvantage of standing heat losses, specific legionella management challenges, and the physical space that they take up in the building.
Part-storage – these include an element of storage together with (typically) direct gas heating that allows the system to meet surge loads of limited duration while also being able to provide a continuous output that meets general, non-peak, requirements. An example of such an appliance is shown in Figure 1, which is able to deliver 440 litres of hot water (with a 50K temperature rise) in the initial 10 minutes, and then sustain a continuous flowrate of 29 litres per minute.
The storage component also provides a limited buffer in case of interruption to the heat supply and, being relatively small, can be readily designed with low heat loss so that the stored hot water may be effectively maintained at the design operating temperature at all times when the building is occupied. An example schematic for such a system is shown in Figure 2.
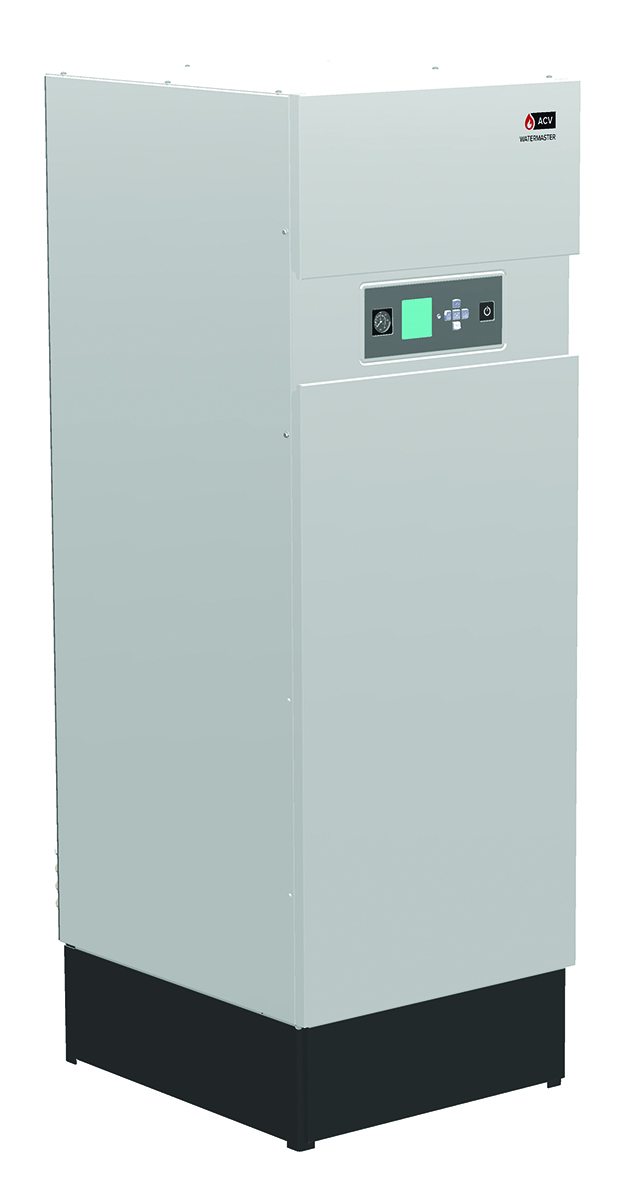
Figure 1: Example of a part-storage gas water heater. This example can provide 440 litres hot water (with a 50K temperature rise) in the initial 10 minutes and then sustain a continuous flowrate of 29 litres per minute (Source: ACV)
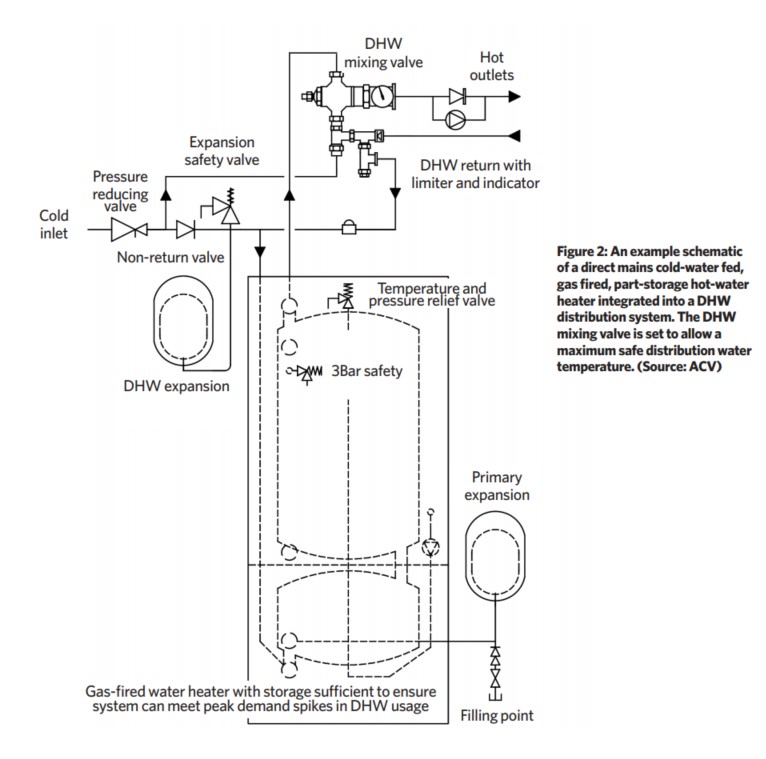
Instantaneous/continuous – these are fed with a direct cold-water supply and are able to continuously supply hot water at anything from 0.2 litres per minute for an above-basin 3kW electric handwash unit, through to ‘multi-point’ heaters (including combi boilers) serving several outlets at 50°C to 60°C, and up to dedicated modular gas hot-water heaters capable of producing, for example, 14 litres per minute of hot water (with a 50K temperature rise) for each wall-hung unit.
Some units are designed to accept returning recirculating DHW as well as a cold-water feed, or potentially preheated – but wholesome – water from other sources.
High-efficiency plate heat exchangers are also used for continuous-flow DHW heaters, with hot water – from a boiler or other source – as the primary fluid and the feed to the secondary wholesome DHW. There are hybrid systems deployed that, for example, employ renewable heat sources or recovered/waste heat to pre-heat or fully heat domestic hot water, together with heat exchangers and thermal stores.
There is no lack of information sources that can be used to size DHW systems. The challenge is selecting the source of information, and estimation method, to provide a reasonable prediction of how a particular building and the expected occupants’ hot-water consumption are best represented.
As discussed in the LUNA project6 stage 1 report published by the team at Heriot-Watt University, there are many different international standards and codes that are designed to provide information to facilitate hot-water system sizing. The methods of modelling vary (see ‘Modelling methods’ boxout), and those commonly employed in the UK have evolved from the same root of a probabilistic modelling technique that was originally based around the concept of the ‘loading unit’ (LU).
The origin of the LU is more than 50 years old (see boxout), but the actual values and application of ‘traditional’ LUs have been developed in an attempt to provide a better prediction of likely water use, principally to reduce oversizing – particularly in larger (non-residential) installations.
Many of the methods used around the world are empirically based and, with the increased maturity and availability of digital modelling techniques, there is a growing body of work employing stochastic procedures (such as that being developed at Heriot-Watt University1).
In the UK, there are four predominant – and potentially confusing – choices for predicting hot-water needs:
BS EN 806-3,7 the British adoption of the European standard, has LUs that have a different basis to traditional LUs and, although numerically similar to the CIPHE ‘low’ category (see right), result in lower predicted hot-water demands when summed and referenced to the method’s conversion chart. This is currently the method that is recommended for supplying single and multiple dwellings.
BS 8558,8 which was written to complement BS EN 806-3 as a means of replacing the long withdrawn BS 6700, maintains the traditional LU method, with LUs that are similar to the CIPHE ‘medium’ classification (see below). When resolved, using the logarithmic conversion chart, the accumulated LUs provide similar hot-water demands to CIPHE (medium). This is the generally recommended method for larger non-residential buildings.
CIPHE4 employs LU values developed from the same probabilistic root as the previous methods but provides more detailed LU discrimination by considering period and frequency of use of each appliance, with three classifications of use: low, medium, and high. This is a popular choice for sizing systems, and provides less diversity in the calculation and therefore less risk for design failure, but potentially increases the opportunity for oversizing.
CIBSE Guide G3 employs the CIPHE LUs for hot-water estimation, and also includes a series of curves to estimate the appropriate size of hot-water storage where a traditional storage system is used.
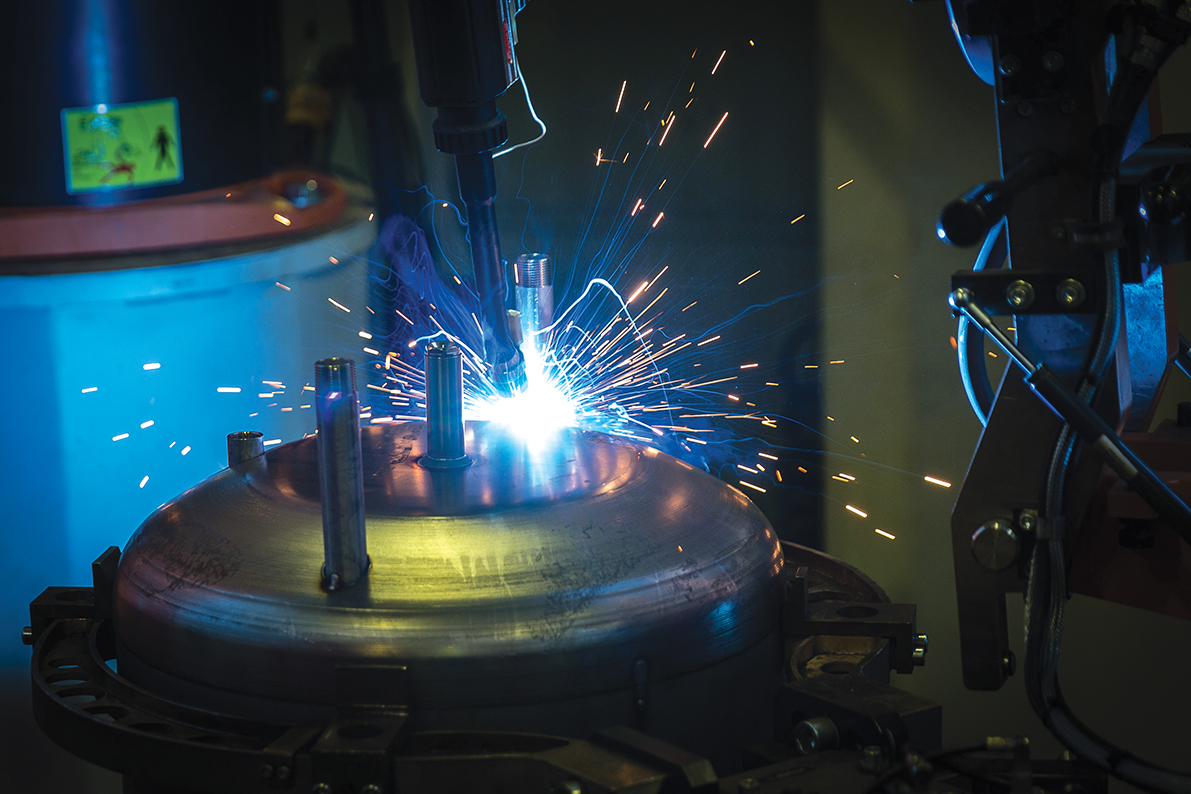
The storage vessel being welded during the manufacturing process (Source: ACV
The current CIPHE and CIBSE guidance were both developed when the bulk storage of DHW was the norm, and newer methods will inevitably evolve in an era where part-storage and instantaneous, continuous-flow systems are becoming more commonplace.
Where there are specific known (empirical) flows and periods of use, then these may be applied directly. For appliances that are known to be likely to have short periods of continuous use at intervals – for example in sports stadia, institutional kitchens, theatres and schools – Whorlow9 explains, with an example, that it is possible to assess the peak simultaneous design flow by reverting to base probability data to meet the normal 99% satisfaction criterion.
Oversizing leads to a number of consequences, which can include oversized plant and distribution equipment; increased opportunities for stagnation in pipework systems (with associated risks of biofilm growth); and increased use of resource with associated financial and environmental impacts.
The LUNA report5 summarises that methods that employ an empirically based statistical framework to offer a better fit with measured data and concludes, on the basis of both statistical validity and a comparison with measured data, that a move away from the use of traditional LUs is now timely.
THE ORIGIN AND DEVELOPMENT OF UK LOADING UNITS
In the 1960s, Harry Howick, past-president of the Institute of Plumbing (IoP, now CIPHE), developed a loading unit system based on the concept of the US fixture unit. This designated relative outlet demand compared to the ‘base appliance’ (a wash basin used every 20 minutes set as one demand unit) and enabled a simplified approach using the theory of probability. A probability graph is used to convert the cumulative load from connected appliances, in a distribution network, into a simultaneous design flow. That original method is reflected in the current BS 85587 and CIPHE4.
(Developed from information in SOPHE TB 16-19)
MODELLING METHODS
Probabilistic models are based on a prescribed probability distribution that associates some measure of likelihood of occurrence with each of the possible outcomes. Most of such models are based on the 1940 US paper by Hunter10 for estimating the 99th percentile of the simultaneous water demand during a busy period. This assumes that water use is a random process, that not all the outlets are used simultaneously, and that estimating simultaneous water demand during a busy period can be modelled within the framework of probability.
Stochastic models are designed to account for randomness and uncertainty, and are often based on Monte Carlo simulation processes that initiate a modelling procedure driven by a set of input parameter values and initial conditions (obtained/collected through some known/measured data or by analysis of information to define various probability distributions).
Stochastic models lead to water-demand profiles corresponding to different sets of input parameter values and initial conditions, allowing various aspects of uncertainty that are not possible with a traditional empirical modelling style that can handle extreme values more effectively than the other modelling schemes.
Empirical modelling approaches (sometimes referred to as ‘deterministic’) use observations to estimate mathematical formulae/expressions. Formulae, charts and tables can be derived by exploring the mathematical/statistical relationships between input and output (measured/observed) data values. In general, most of the standards for the sizing of pipework are based on empirical approaches.
(Developed from information in LUNA stage 1 report6)
© Tim Dwyer, 2021.
■ Turn to page 78 of CIBSE Journal, May 2021, for further reading and references.