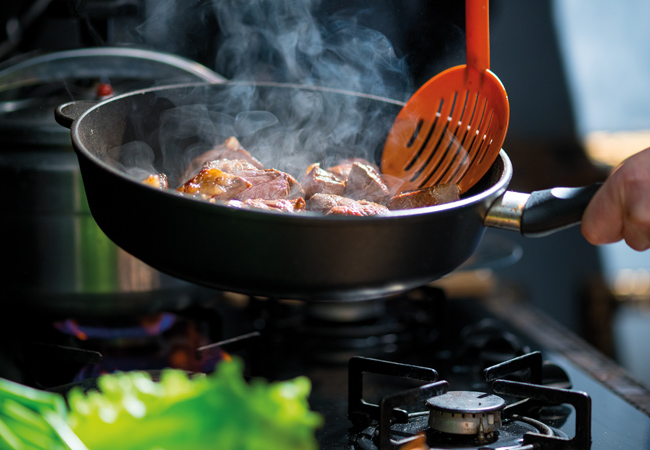
Cooking generates high concentrations of particulate matter (PM), nitrogen oxides (NOX) and carbon monoxide (CO). Emission rates depend on the cooking method, and oil and food types'
In developed countries, we spend approximately 90% of our time indoors, and much of that in our homes1. Exposure to air pollutants, therefore, happens almost exclusively indoors – even for pollutants generated outdoors, which come in via windows, doors and cracks in buildings.
Activities such as cooking and cleaning can produce high pollutant concentrations, with potential impacts on human health, including respiratory and cardiovascular diseases2,3. However, environmental regulation still focuses on outdoor air quality, with the exception of specific occupational exposures4.
A joint project between the universities of York, Chester and Nottingham has studied the production of different chemicals during cooking and cleaning. The Impeccable (Impacts of cooking and cleaning on indoor air quality: towards healthy buildings for the future) project used state-of-the-art equipment, coupled with computational modelling11 to understand the complex underlying chemistry that occurs during and after domestic cooking and cleaning activities.
The study recommends the most effective ventilation strategies to reduce the air pollution created by cooking and cleaning.
The climate emergency has forced us to think more carefully about energy efficiency. Buildings are becoming increasingly airtight, but we have the potential to increase our exposure to indoor air pollution if sources such as cooking and cleaning dominate our personal exposure to air pollution.
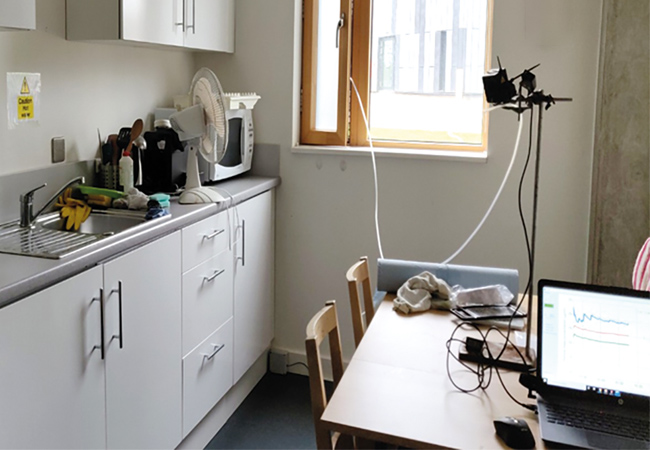
Measuring VOCs while cooking in the home lab
We need to understand the sources and reactions of pollutants indoors, particularly as an increasing weight of evidence shows that secondary pollutants are more harmful to health than primary emissions9. For instance, the carcinogen formaldehyde is a reaction product of limonene oxidation, the latter species being a key component of many cleaning formulations10.
Cooking generates high concentrations of particulate matter (PM), nitrogen oxides (NOX) and carbon monoxide (CO). Emission rates depend on the cooking method, and oil and food types.
Emissions of PM are higher for frying than boiling and steaming, and higher when you fry meat compared with vegetables2. During cooking, PM concentrations can reach several hundred mg/m3, particularly when frying2, exceeding acute health standards over several hours5. Cooking can also generate ultra-fine particles (< 100 nm in diameter), which are associated with adverse effects on the respiratory and cardiovascular systems6.
Cleaning is another regular activity indoors, with bleach cleaning having a large impact on gas-phase and surface chemistry7. The prevalence of asthma among domestic cleaning staff suggests that cleaning activities may cause adverse health effects8.
The Impeccable project
The experiments undertaken range from benchtop ones in a lab to determine specific emission rates from individual products or processes, through to more realistic settings, where full meals are cooked in a more home-like environment.
Measurements are taken using on- and off-line diagnostics, to determine types and quantities of various indoor air pollutants. Selected-ion flow-tube mass spectrometry (SIFT-MS) was used to measure real-time concentrations of more than 40 volatile organic compounds (VOCs) during the cooking and cleaning experiments.
This technique uses a plasma to generate ions, which then ionise VOCs present in the sample gas12. These charged species are then separated and quantified based on their mass-to-charge ratios. An example of the real-time data obtained during a cooking experiment is shown in Figure 1.
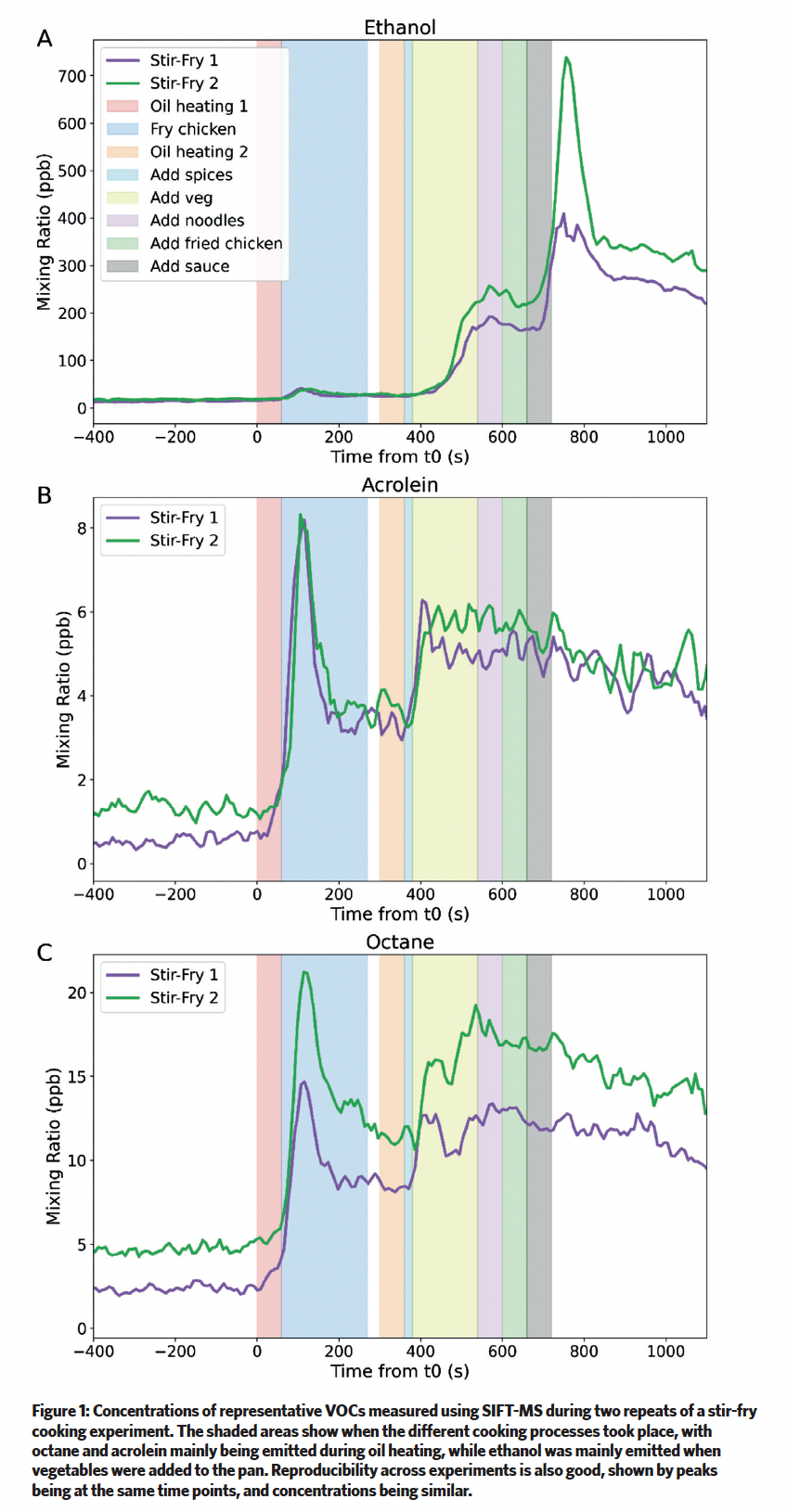
The modelling of indoor air chemistry has been carried out using INCHEM-Py, an open-source box model that uses a detailed chemical mechanism, combined with parameterisation for indoor-outdoor exchange, gas-particle partitioning, surface deposition, and internal photolysis13.
The detailed model allows us to simulate the time-evolved concentrations not only of the species emitted from indoor activities, but also of those formed after chemical reactions, such as formaldehyde, peroxyacylnitrate species (PANs) and PM.
The simulated concentrations of some secondaries formed after the typical stir-fry cooking experiment are shown in Figure 2. These products are often more harmful to human health than the original emissions, so understanding how they are produced can help inform scientifically rigorous mitigation measures (such as extraction of cooking fumes or increased ventilation).
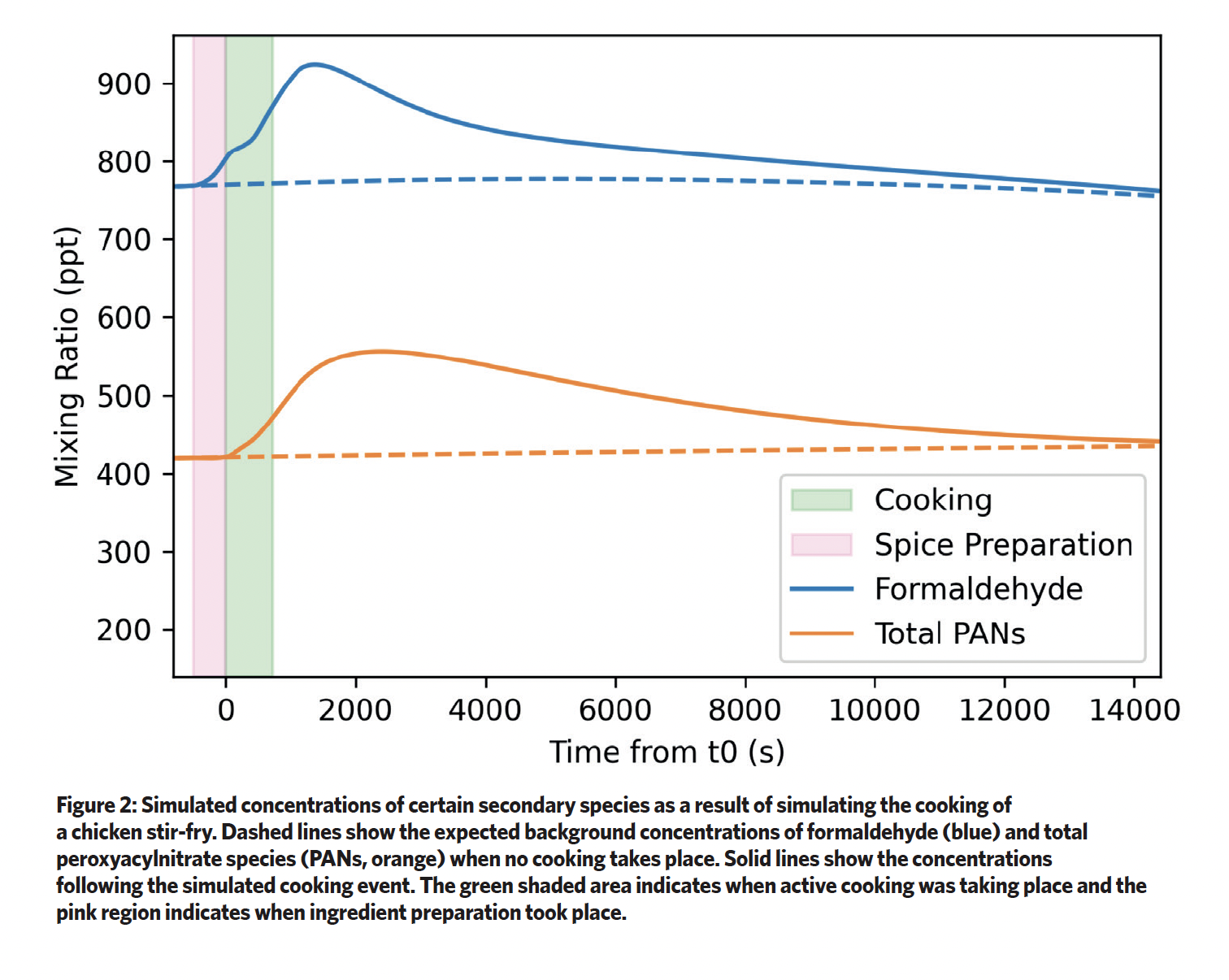
Relevance for building design and operation
Kitchen ventilation is regulated by Approved Document F of the Building Regulations in England. In new houses, there is a requirement for a cooker hood that extracts to the outside or a wall-mounted fan.
The advantage of the cooker hood is that it captures cooking contaminants before they mix in the kitchen, whereas the wall fan allows the contaminants to mix before they are extracted. The required flowrate through the hood is half that of the fan, which implies that it should capture 50% of all emitted contaminants. This is known as a capture efficiency. There is no requirement to test hoods to make sure they meet this requirement, although there is now an ASTM standard14 that tests some types of hood in a laboratory environment. Future versions of Approved Document F should require cooker hoods to conform to the ASTM standard or allocate a punitive capture efficiency to those that are untested.
Ventilating for 10 minutes after cooking has finished has a significant effect on exposure and should be recommended by public health campaigns15,16. The higher airflow rate through the wall fan means that it reduces the concentration of contaminants in the air faster than the hood. So having multiple flowrate settings on a hood is advantageous. The best place to locate a hood is against a wall, between cabinets, directly over the gas burners/hob, and as close to the burners as the manufacturer allows. Noise is often a barrier to their use, so using short, wide, straight, rigid, noise-absorbing ducts that reduce air velocity to 2-3 m/s is best practice.
When cooking, using the back burners increases the pollutant capture efficiency16. Consider using the hood, at least on low speed, for general kitchen ventilation when using other appliances, such as toasters.
Using induction rather than gas burners, and electric rather than gas ovens, reduces the emission of nitrogen dioxide (NO2), but the chronic harm from exposure to NO2 is significantly less than that from fine PM. A good cooker hood will capture NO2 from a burner, so using the hood is more important than changing from a gas to an induction stove.
In very airtight homes (<1 m3/h/m2) make-up air into the kitchen or home is needed and this can be provided using mechanical ventilation with heat recovery (MVHR). Reclaiming thermal energy from the exhaust stream can be problematic in MVHR systems because grease can clog the heat exchanger. This problem has yet to be solved and may require more frequent cleaning of filters.
Some homes have recirculating fans, which pass air through a particle filter and resupply it into the kitchen, rather than exhaust it outside. In this instance, it is imperative to use induction hobs. Electric hobs have their own complications because they can emit PM when they get dirty. For homes that have no fans in their kitchens, windows and doors should be opened when cooking and cleaning to provide ventilation in the short term. In the medium term, a mechanical system should be installed and trickle ventilators kept open at all times.
We would like to thank EPSRC for funding for the Impeccable project (EP/T014474/1).
About the authors
Professor Nicola Carslaw is professor of indoor air chemistry in the Department of Environment and Geography at the University of York
Dr Helen Davies is research associate in chemistry in the Department of Environment and Geography at the University of York
Dr Benjamin Jones associate professor in the Department of Architecture and Built Environment at the University of Nottingham.
References
- Nazaroff, W. W.; Goldstein, A. H., (2015), Indoor Air, 25, (4), 357-61
- Abdullahi, K.L. et al. (2013), Atmos. Environ. 71, 260-294
- Wolkoff, P. (2013) Int. J. Hygiene Env. Health, 216, 371-394
- Weschler, C.J., and N. Carslaw, Environ. Sci. Technol., 2018, 52, 2419-2428
- Logue, J.M., et al. (2011), Indoor Air, 21, 92-109
- Rim, D. et al. (2013), Environ. Sci. Technol., 47, 1922-1929
- Wong, J. P. S et al. (2017) Indoor Air, 27, 1082-1090
- Medina-Ramón, M. et al. (2006) Occupational and Environmental Medicine, 62, 598-606
- Buchanan, I.S.H. et al. (2008), Indoor Air 18, 144-155
- Wang, C., M., et al. Environ. Sci. Proc. Impacts, 2017, DOI: 10.1039/C6EM00569A
- Impeccable website: impeccable.york.ac.uk
- Smith, D., Španel, P., (2005), Mass Spectrometry Reviews, 24, 661-700
- Shaw et al. (2021). Journal of Open Source Software, 6(63), 3224, doi.org/10.21105/joss.03224
- ASTM, Measuring Capture Efficiency of Domestic Range Hoods. Report. 2018.
- Holgate S et al. The inside story: Health effects of indoor air quality on children and young people. Royal College of Paediatrics and Child Health; 2020.
- O’Leary C, et al. Setting the standard: The acceptability of kitchen ventilation for the English housing stock. Building and Environment. 2019:106417.