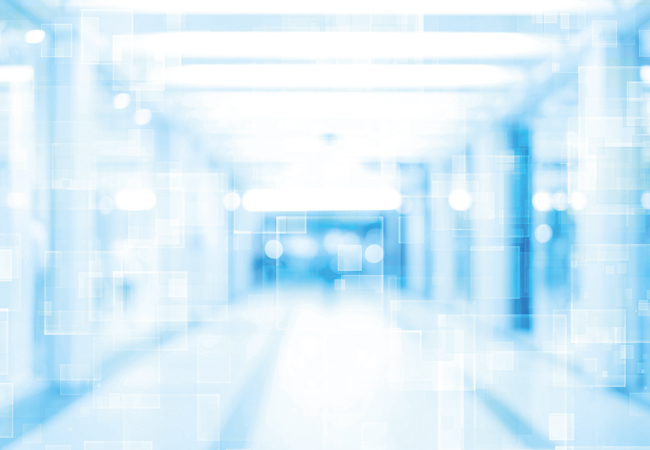
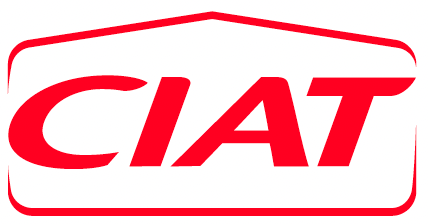
Indoor air quality (IAQ) in hospitals will significantly impact occupant health and wellbeing. Aside from the more recent impacts of the spread and containment of SARS-CoV-2 in healthcare facilities, there are many other airborne pollutants that, together with the air temperature and humidity, will determine hospital IAQ. This CPD will explore some of the key variables that combine to provide the ‘all-inclusive’ IAQ.
In England alone, as well as providing a safe environment for the 35 million-plus patient bed-days, hospital air is shared by approximately one million health workers. As indicated in Figure 1 (overleaf), the sources that contribute contaminants to the indoor air are numerous and often interrelated – and these will affect the quality of the environment for patients, staff, and visitors.
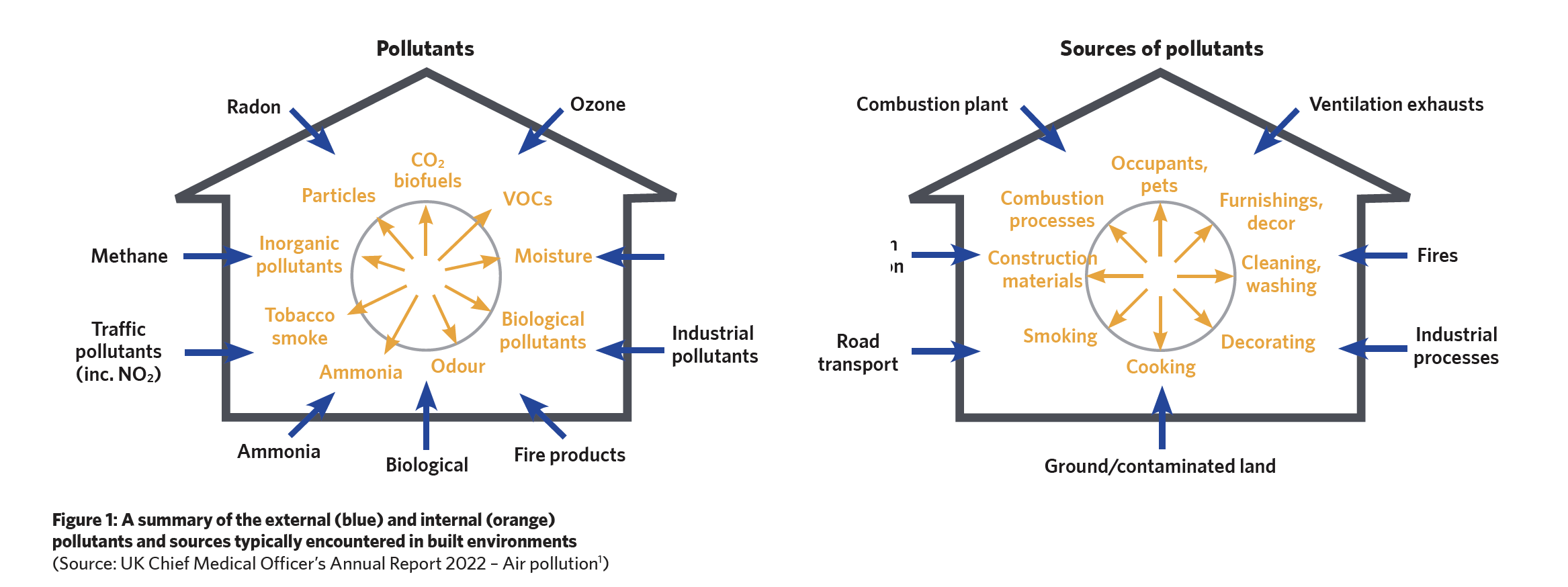
In spaces that have at least some ventilation, CO2 is unlikely to reach levels that cause any health concerns. A CO2 limit is often set in the design specification as a proxy for the sufficiency of ventilation. However, the recent review2 by Fonseca et al notes that it can be challenging to develop ventilation strategies to address the different simultaneously-occurring indoor air pollutants with the formation of secondary pollutants from the reaction between the primary pollutants, as well as with other chemicals or those introduced by ventilation.
In healthcare settings there are many microorganisms – bacteria, viruses, parasites and fungi – that are potentially airborne and can lead to ‘nosocomial infections’ – otherwise known as healthcare associated (or acquired) infections (HAI). A study by Guest et al,3 based on pre-Covid 2016-17 data, estimated that there were 834,000 cases of HAIs annually in the NHS in England. The proportion of infections that can be directly associated with the IAQ and the airborne load is unknown – and likely impossible to establish.
The 2020 review paper4 by Hiwar et al notes that IAQ parameters – such as temperature, relative humidity, CO2 level, particle mass concentration, and particle size – are, in any case, important for the comfort, health and wellbeing of those in hospitals, and will likely impact the ‘bioburden’ in the environment. This relates to the whole hospital population – Fonseca et al noted2 that headaches, fatigue, dryness and irritation of the eyes and skin are common complaints of healthcare professionals, which are often associated with poor IAQ.
Air temperature and particularly humidity are linked with the survival of microorganisms, with many bacteria and fungi favouring more humid conditions and some evidence of virus survival increasing at lower humidities (typically below 40% relative humidity). Low relative humidity, as is typically encountered in heated healthcare premises in winter, also keeps large droplets suspended in air for extended periods of time.5
In this context, the term ‘aerosol’ is widely applied and is variously defined but commonly understood to have a characteristic dimension smaller than 5μm. As explored by Randall et al,6 there are various definitions of ‘aerosols’, with most contemporary sources relating the 5μm threshold to particles that remain suspended in the air for longer periods. However, he explains that this distinction is erroneous, and is actually based on what reaches deepest in the lungs. This has likely confused the understanding of the droplet and aerosol transmission mechanisms as defined by a 5μm threshold.
As well as the much-investigated SARS-CoV-2, there are many other airborne microorganisms that remain viable in the air in an ‘aerosol’ state.7 The stability of viruses in aerosols is affected by relative humidity through several physical mechanisms, but the role of these mechanisms in virus inactivation is not fully understood. Hiwar’s review found conflicting results linking IAQ parameters and microorganism prevalence and survival, with any relationship being dependent on the specific microbe.
Typically, the pollutants in the outdoor air that impact occupant wellbeing are the nitrogen oxides, ozone, sulphur dioxide, and particulates. As noted by the Federation of European Heating, Ventilation and Air Conditioning Associations (REHVA),8 particle size distribution may be expressed in different ways, according to their number, surface, and volume.
The UK Air Quality Expert Group (AQEG)9 indicates that PM10 corresponds to the ‘thoracic convention’ – the size fraction of inhaled particles that penetrate beyond the larynx, while PM2.5 corresponds to the ‘high risk respirable convention’ – the size fraction that penetrate to the unciliated airways (essentially to the gas-exchange surfaces) and is of particular concern for high-risk groups (children, the elderly and infirm). Most particles in typical urban air are ultrafine particles (UFP), with at least one dimension smaller than 0.1μm – these are known as PM0.1.
However, most of the mass of airborne particulate matter is associated with particles larger than 0.1μm – this consideration is particularly relevant when evaluating the collection efficacy of air-cleaning devices that is typically undertaken by measuring either particle count or particle mass. UFP penetrate deep into the respiratory system, allowing interactions with lung tissue and potentially passing into the blood stream. This, together with the hypothesis that toxicity of particulate matter is governed by the surface area of the particles rather than their mass, has led to suggestions that UFP may be particularly harmful to health.9
As noted in the 2020 report10 by the Royal College of Physicians 2020, ventilation may also lead to the introduction of high ozone (O3) concentrations. O3 in the troposphere (ground level) is noted as a toxic air pollutant produced by photochemical reactions from nitrogen oxides and volatile organic compounds (VOCs).11 Ozone is very reactive, and can produce secondary pollutants from chemical reactions, including with d-limonene (used in air fresheners, for example). With enough ventilation, the conditions for chemical reactions are disrupted, and any secondary pollutants created would be quickly flushed out of the building. However, short term, high levels of O3 are associated with coughing, asthma attacks, and acute respiratory infections and failure, particularly in those with lung diseases.12
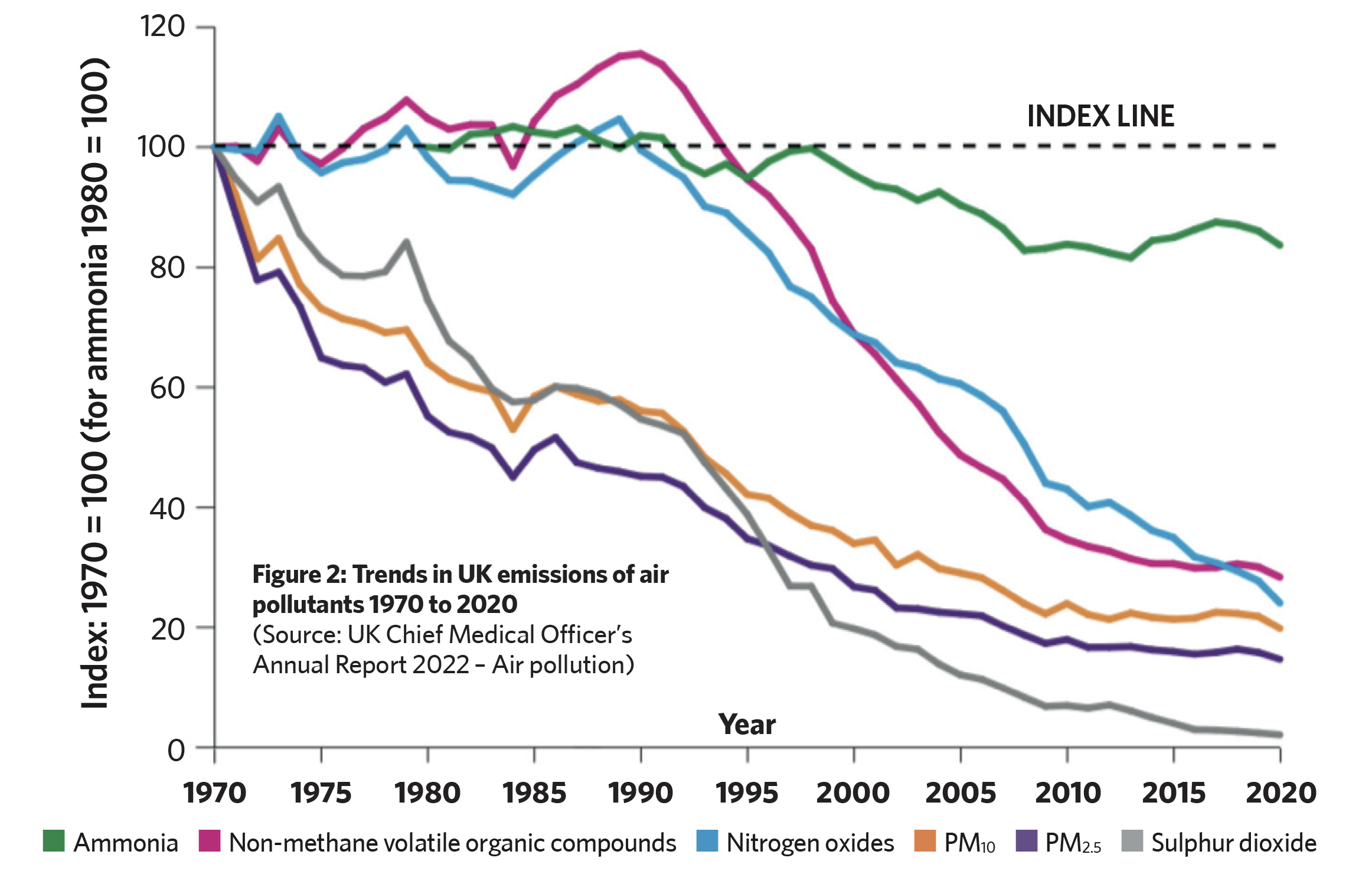
As recently noted by the chief medical officer,1 with the decrease in outdoor air pollution – as observed in some UK environments (as illustrated in Figure 2) – the significant focus should be on employing suitable ventilation strategies to dilute and remove those contaminants that are emitted from within healthcare premises. Occupant activities within hospital settings – such as talking, sneezing, coughing, and movement – contribute to the release of airborne biological contaminants, including particulate matter, chemical pollutants, and bioaerosols.
The review13 by Ibrahim et al noted that the use of medical equipment, such as nebulisers and disinfection devices, can introduce pollutants into the indoor environment. Likewise, polymer materials, electronic devices, and medical supplies made from specific materials can emit harmful compounds, impacting IAQ. For instance, plastic products may release phthalates, and electronic devices used for administrative purposes may emit VOCs such as benzene, toluene, ethylbenzene, and xylene.
The Covid-19 pandemic demonstrated – and the UK AQEG9 confirmed – that ventilation is not just about bringing in fresh air. Inappropriate ventilation can spread airborne infections from one bed to another and from one ward to another.
Therefore, the role of well designed, maintained and operated ventilation is fundamental to reducing unavoidable indoor air pollutants and preventing pathogen transmission, and also minimising the introduction of externally sourced pollutants. It can often be designed to mitigate some of the overheating challenges from climate change, while holistically considering energy consumption and consequent carbon emissions.
And while appropriate mechanical ventilation may effectively curb ‘bioaerosol levels’, research14 reveals that persistent contamination of hospital indoor air by fungi can still cause incidences of nosocomial opportunistic mould infections, which can cause localised infections, fatal diseases, allergic responses, and be life-threatening in immunocompromised patients.
The specific focus on IAQ can quickly change in healthcare premises, as witnessed during the Covid pandemic – which sparked a surge in studies examining the efficacy of air quality control measures. Multiple published works have attempted to categorise methods, systems and practices that are employed to eliminate, or at least control, virus size particles in the occupied spaces.
This would typically encompass virus sizes of approximately 0.1μm and a (coughed) respiratory droplet size of 1μm and above. The continued research will undoubtedly inform emerging challenges in air quality control. For example, the impact of ultrafine particles that can penetrate deep into lung passageways and enter the bloodstream – is still being uncovered, but there is already a solid body of evidence15 that links short periods of exposure to ultrafine particles to significant adverse health effects.
Emerging fungal pathogens and infections pose increasing threats to public health, and most at risk are those with a compromised immune system. A recent study16 notes that the vast majority of the world’s potentially pathogenic fungi do not do well at body temperatures. However, it has found that raised temperatures, as may be experienced with climate change, cause the fungus Cryptococcus deneoformans – associated17 with a quarter of a million cases of meningitis each year – to accelerate in its ability to adapt, and so potentially present an increasing pathogenic challenge for IAQ control. This is undoubtedly only one of many pollutants that will be exacerbated by climate change.
The array of available ventilation systems, as generically illustrated in Figure 3, whether mechanical or natural, play an integral role in maintaining appropriate IAQ. In the review by Ibrahim,13 mechanical ventilation emerges as the more potent means of reducing total bioaerosol levels, surpassing the effectiveness of natural ventilation. Also, centralised ventilation systems exhibit enhanced control over chemical and particle contaminants compared with non-centralised counterparts.
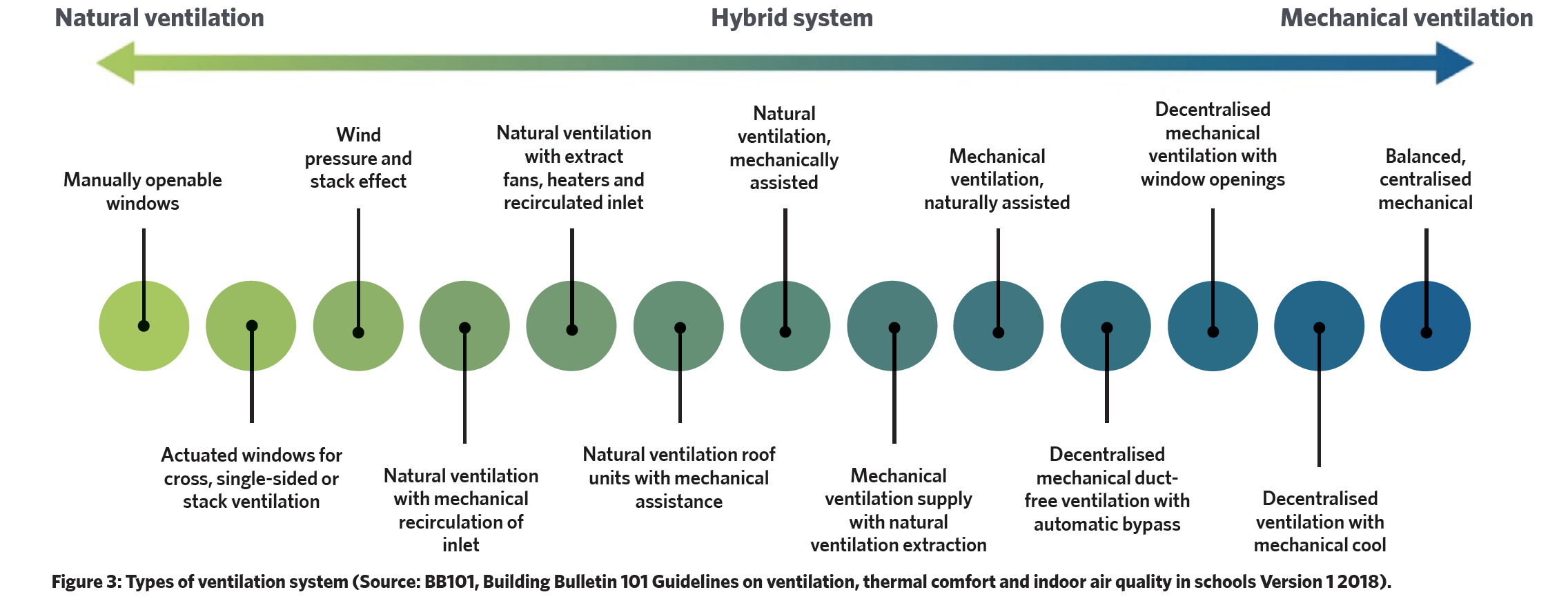
A key UK reference that guides the ventilation (and IAQ) requirements is the freely downloadable NHS England publication Health Technical Memorandum 3 – Specialised ventilation for healthcare premises (HTM03). This two-part extensive and detailed HTM provides guidance in terms of performance specifications and methods to manage and maintain appropriate conditions for the health and welfare of occupants, and for processes such as centralised food preparation facilities.
Its core principle is that the default method of ventilation should, as far as possible, be natural ventilation followed by mixed mode (natural with mechanical ventilation), with mechanical ventilation being the last option. It notes that odour control is likely to be the dominant reason for providing ventilation where, in the absence of other guidance, 10L.s-1 per person should be taken as the minimum ventilation requirement. In clinical areas, mechanical ventilation systems would normally provide ‘full fresh air’, and in other areas typically a minimum 20% outdoor air.
HTM03 notes that filtration will be required to remove airborne particulate contamination and, if necessary, odours. In treatment and support areas, the overriding requirement may be for airborne infection control, hazard containment, the stability of specialist equipment, or relate to a specific department’s function. The standard of filtration will depend on the activities within the occupied spaces and, except for special areas, the filtration is only required to maintain hygienic conditions for the health and welfare of occupants and processes; protect finishes, fabrics and furnishings; and to protect the supply air system.
HTM03 concurs that in normal situations viable particles will most likely originate from the occupants of a space and not from the incoming air, so advises a modest filtration requirement for general areas, with more stringent requirements for more critical areas or when outdoor air conditions are particularly polluted. Efficiency or high-efficiency (EPA or HEPA) levels of filtration are only normally required in ultra-clean systems and designated ‘clean rooms’.
Noting that controlling humidity is expensive, the HTM recommends that room air humidity should normally be kept below 70% in order to minimise risks associated with condensation and mould growth. There is no lower limit in typically unoccupied spaces, although some equipment may require closer control.
Although there are basic IAQ requirements that must be met for comfort and health, the demands in healthcare settings can change quickly as a result of unexpected events such as pandemics; changes in transportation, industrial and commercial activities; and the impacts of climate change. It is important for healthcare designers and operators to understand the principles and practices of IAQ control for their specific applications. This will ensure that IAQ systems are properly maintained, and aid in quick and effective developments to meet future challenges.
- UK Chief Medical Officer’s Annual Report 2022 – Air pollution.
- Fonseca, A et al, Indoor Air Quality in Healthcare Units – A Systematic Literature Review Focusing Recent Research, Sustainability 2022.
- Guest, JF et al, Modelling the annual NHS costs and outcomes attributable to healthcare-associated infections in England, BMJ Open 2020;10:e033367. doi:10.1136/ bmjopen-2019-033367.
- Hiwar, W et al, What is the relationship between indoor air quality parameters and airborne microorganisms in hospital environments? A systematic review and meta-analysis, Indoor Air. 2021;31:1308–1322.
- Božic, A and Kanduc, M, Relative humidity in droplet and airborne transmission of disease, J Biol Phys. 2021, 10.1007/s10867-020-09562-5.
- Randall, K et al, How did we get here: what are droplets and aerosols and how far do they go?….., Interface Focus 11: 20210049. bit.ly/CJOct23CPD1.
- Beggs, C, The Airborne Transmission of Infection in Hospital Buildings: Fact or Fiction? Indoor Built Environment 2003.
- REHVA Guidebook 11, Air filtration in HVAC Systems, REHVA 2020.
- Ultrafine Particles (UFP) in the UK, UK Air Quality Expert Group, 2018.
- The inside story: Health effects of indoor air quality on children and young people, Royal College of Physicians 2020.
- bit.ly/CJOct23CPD2 – accessed 31 August 2023.
- bit.ly/CJOct23CPD3 – accessed 31 August 2023.
- Ibrahim, F et al, Hospital indoor air quality and its relationships with building design, building operation, and occupant-related factors: A mini-review, Public Health 2022 doi: 10.3389/fpubh.2022.1067764.
- Sham, NM et al, Fungus and mycotoxins studies in hospital environment: a scoping review, Build Environ. 2021.
- Schraufnagel, DE, The health effects of ultrafine particles, Exp Mol Med 52, 311–317 (2020). bit.ly/CJOct23CPD4.
- Asiya Gusa et al, Genome-wide analysis of heat stress-stimulated transposon mobility in the human fungal pathogen Cryptococcus deneoformans, Proc of the Nat Acad of Sciences, 2023; 120 (4) DOI: 10.1073/pnas.2209831120.
- bit.ly/CJOct23CPD5 – accessed 28 August 2023.