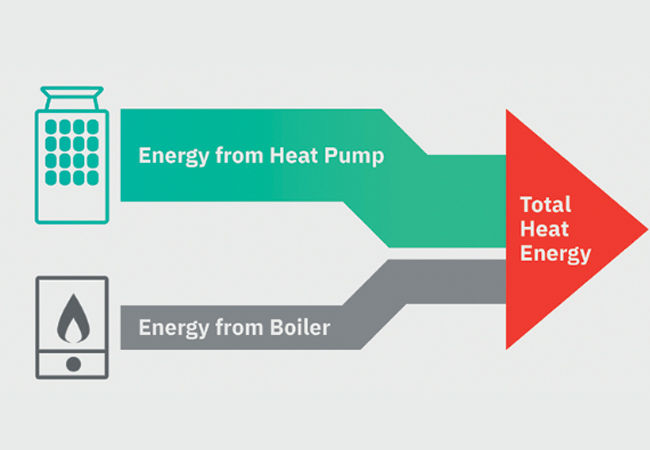
Decarbonising our existing building stock is both a huge challenge and a huge opportunity.
When considering an existing gas-fired heating system that is operating with relatively high water flow temperatures of
70-80oC –compared with today’s typical designs of ≤55oC – application of an electrically driven alternative to match those flow temperatures is fairly straightforward.
Managing the impact on operational costs and capital expenditure (capex) is much more complex, however, especially given the disproportionate cost per kWh of gas vs electricity currently, and the relatively high capex cost of technologies, such as heat pumps, compared with fossil-fuel alternatives.
This is where a bivalent approach can become attractive and possibly help bridge some of these gaps. Focusing on using heat pumps as part of this bivalent solution, we can consider two broad types of configuration: bivalent in parallel and bivalent changeover (see Figure 1).
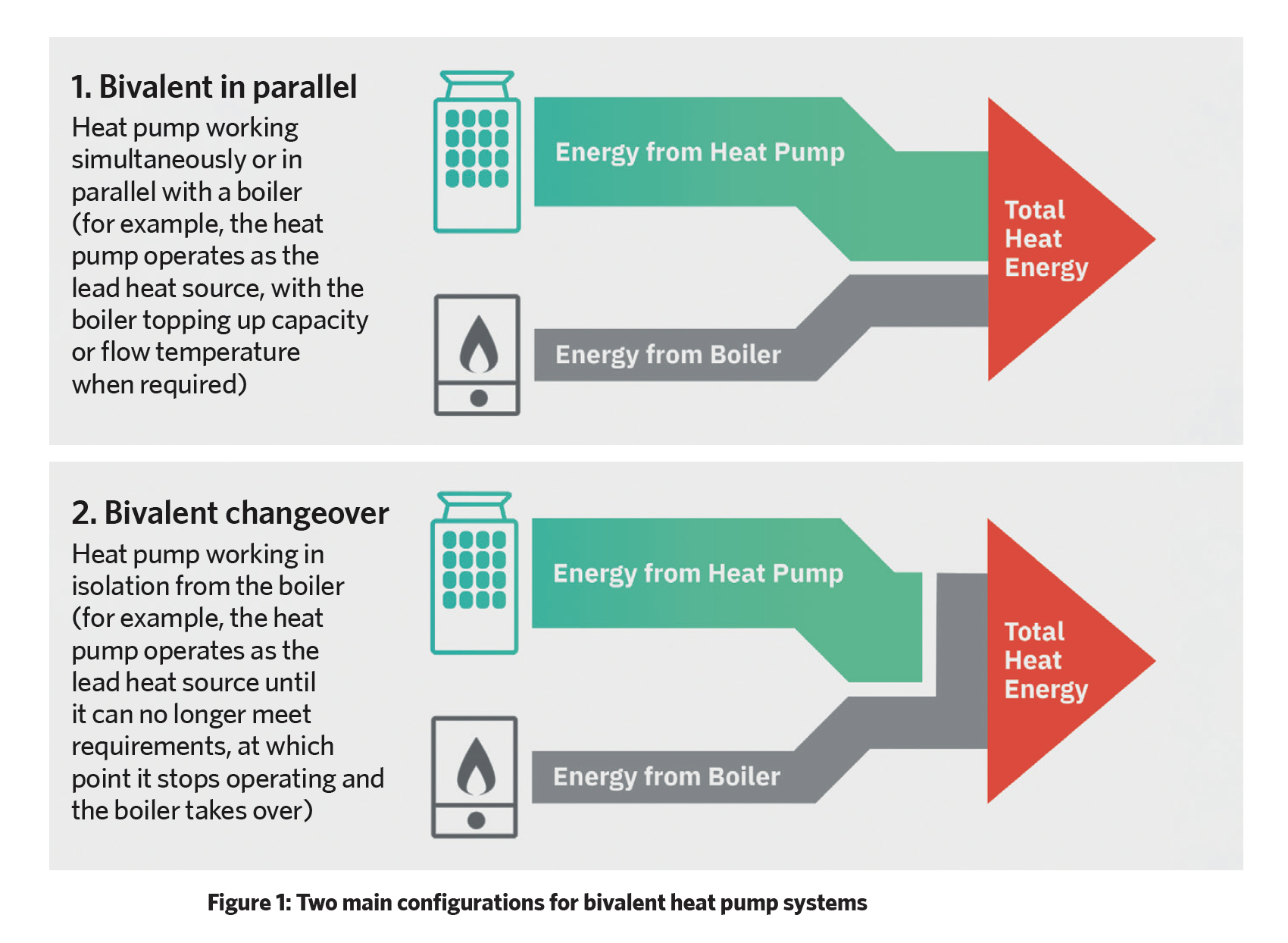
It is typical in the UK for peak space heating capacity (100% load) to only be required for a small number of hours in the year and, therefore, this makes up a relatively small amount of the total kWh of heat energy delivered. This opens up the possibility of deliberately undersizing your heat pump, in comparison with peak load, and only operating it for certain parts of the year, allowing the gas boiler to provide the extra peak capacity or peak flow temperatures when needed. This approach can help reduce upfront costs and plant space requirements.
We know that operating a heat pump at the lowest possible flow temperature and the warmest source temperature will usually deliver the highest efficiency and lowest operating cost. But we must also consider how any heat generated is emitted into the building – this is where the infrastructure of the existing system begins to influence the design approach.
The existing heat emitters (fan coils, air handling unit coils, radiators, and so on) must be assessed to understand their deliverable output capacity at different mean temperatures. This will demonstrate what mean temperature is needed at certain ambient conditions to deliver the required capacity. This is a key piece of information needed to model bivalent parallel and bivalent changeover configurations.
Bivalent changeover configuration:
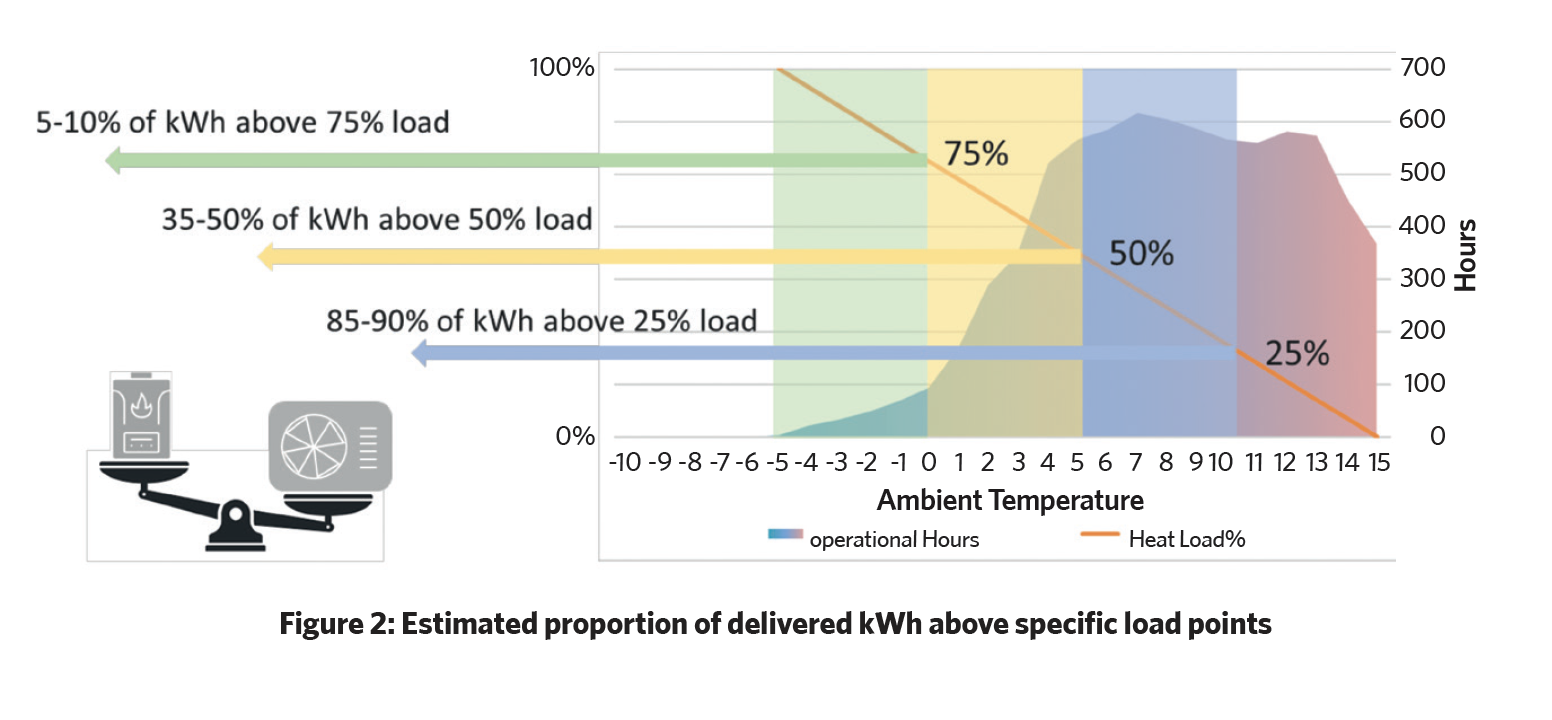
In a bivalent changeover arrangement, the heat pump is deliberately designed not to deliver the peak flow temperature or capacity of the heating system. It will only operate up to a temperature and capacity chosen to match the heat-emitter capabilities and building load at that changeover point.As a result, the heat pump will operate in isolation from the boiler, providing heat to the existing heat emitters until its maximum flow temperature and capacity are reached. At this point, it will turn off and the boiler will take over, delivering the higher-temperature water and increased capacity required to meet the increasing building load.
This arrangement will deliver a lower proportion of annual space heating load from the heat pump compared with bivalent parallel. However, as the heat pump will not operate at peak design conditions, or be asked to deliver high flow temperatures, it will benefit from increased efficiency, resulting in a lower operating costs.
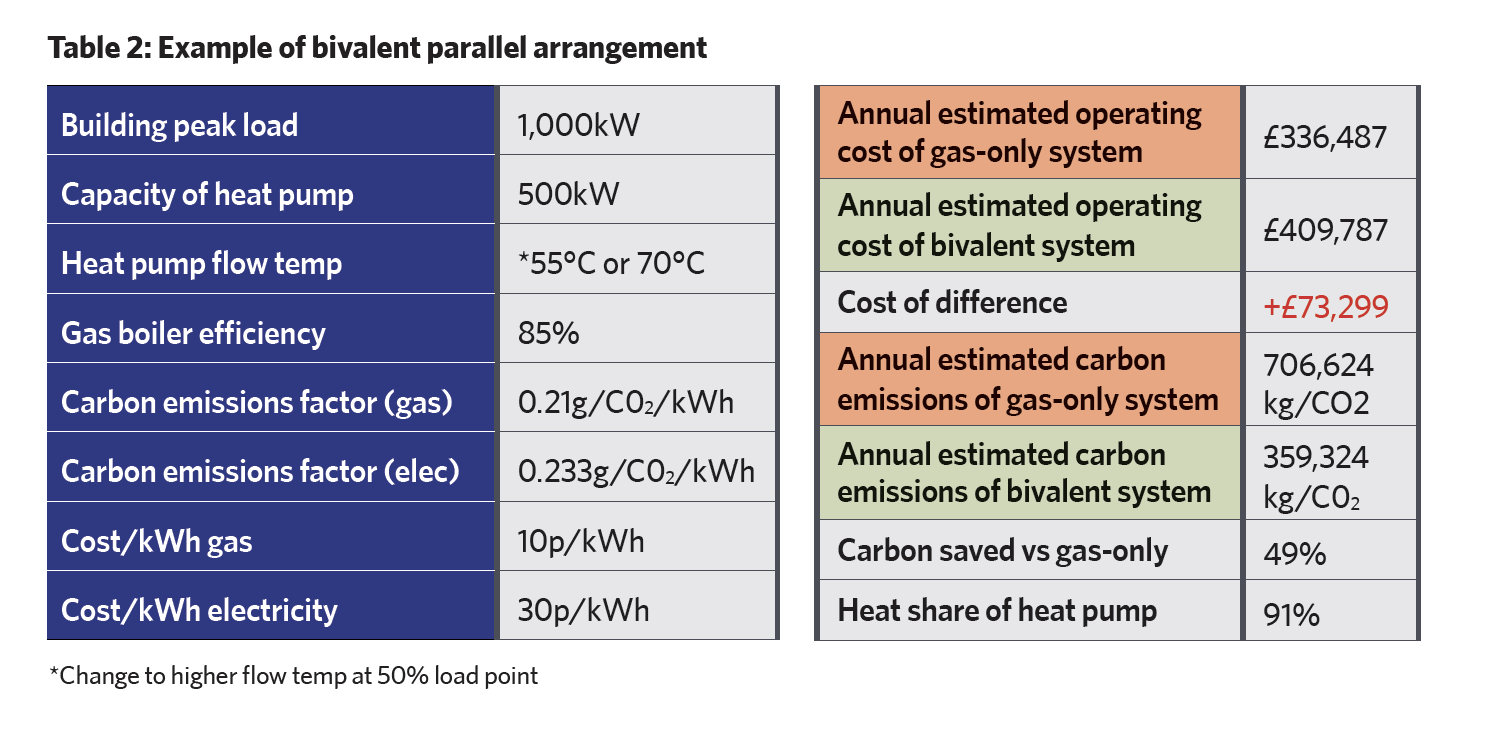
To maximise the ratio of kWh contribution from the capacity of heat pump provided, our research shows the optimum will be approximately 50-75% of the building peak load, with the maximum flow temperature being approximately 55oC. Depending on overall system design and existing heat-emitter capabilities, other combinations can also deliver good results. See Table 1 for an example of a bivalent changeover arrangement. Examples are modelled on the Mitsubishi CAHV-R450 YA-HPB.
Bivalent parallel configuration:
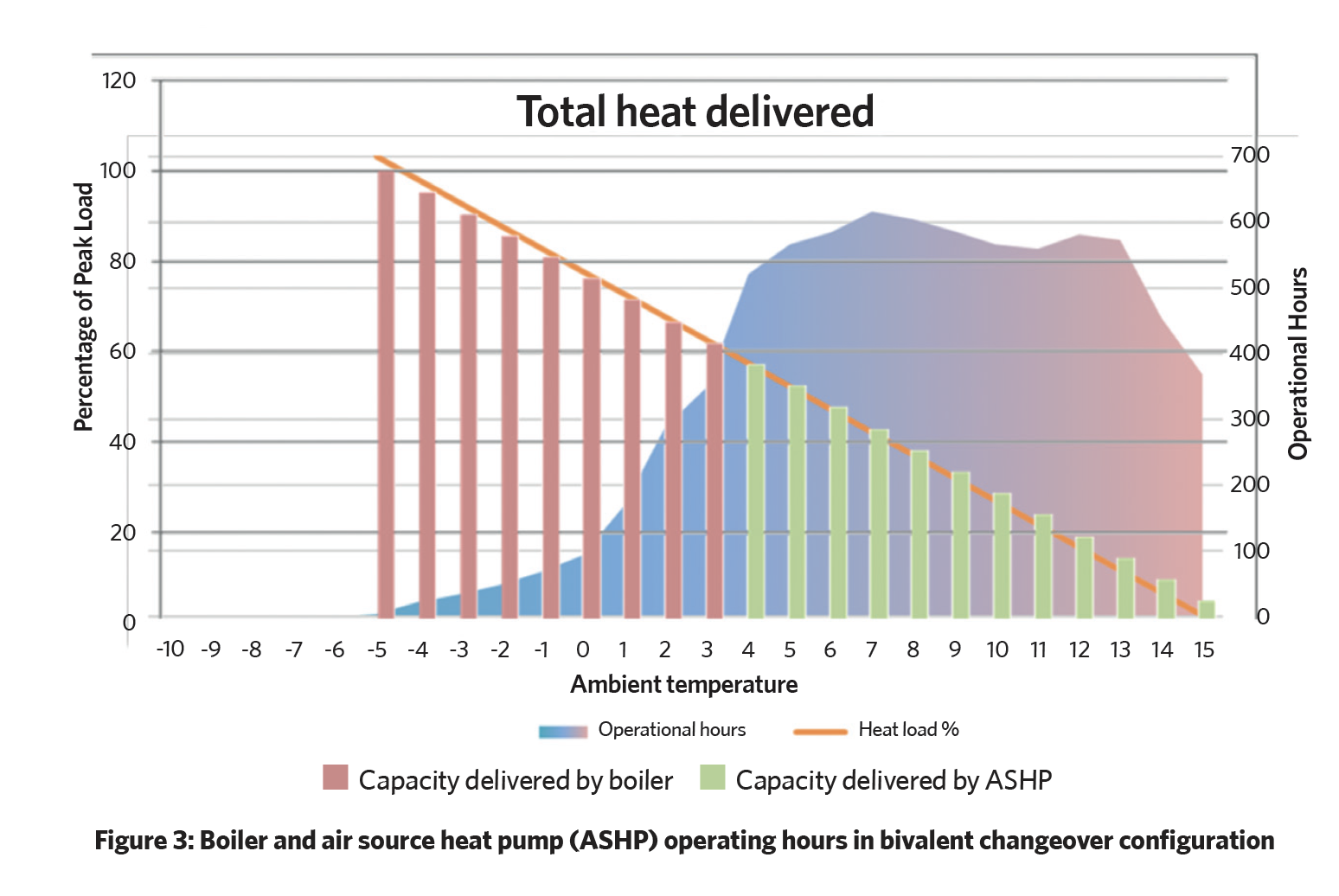
This requires the heat pump to be capable of delivering the peak flow temperature of the system, allowing it to work side by side with the boiler at any time of the year. This means the heat pump can be sized to any capacity and, as Figure 4 shows, deliver heat energy on its own when it has the capacity, or in conjunction with the boiler when the load is greater than the heat pump capacity (the example shows a heat pump sized at 50% of peak load). Flow temperature can be fixed or weather-compensated, but the key design principle is that the heat pump is able to deliver the required flow temperature to meet peak heating demands via the existing heat emitters.
This arrangement will deliver a large proportion of annual space heating load from the heat pump, and probably result in the lowest overall carbon emissions. However, operating the heat pump at potentially high flow temperatures and low ambient conditions will reduce its efficiency, so this configuration will probably result in an increased operational cost compared with the gas boiler-only system.
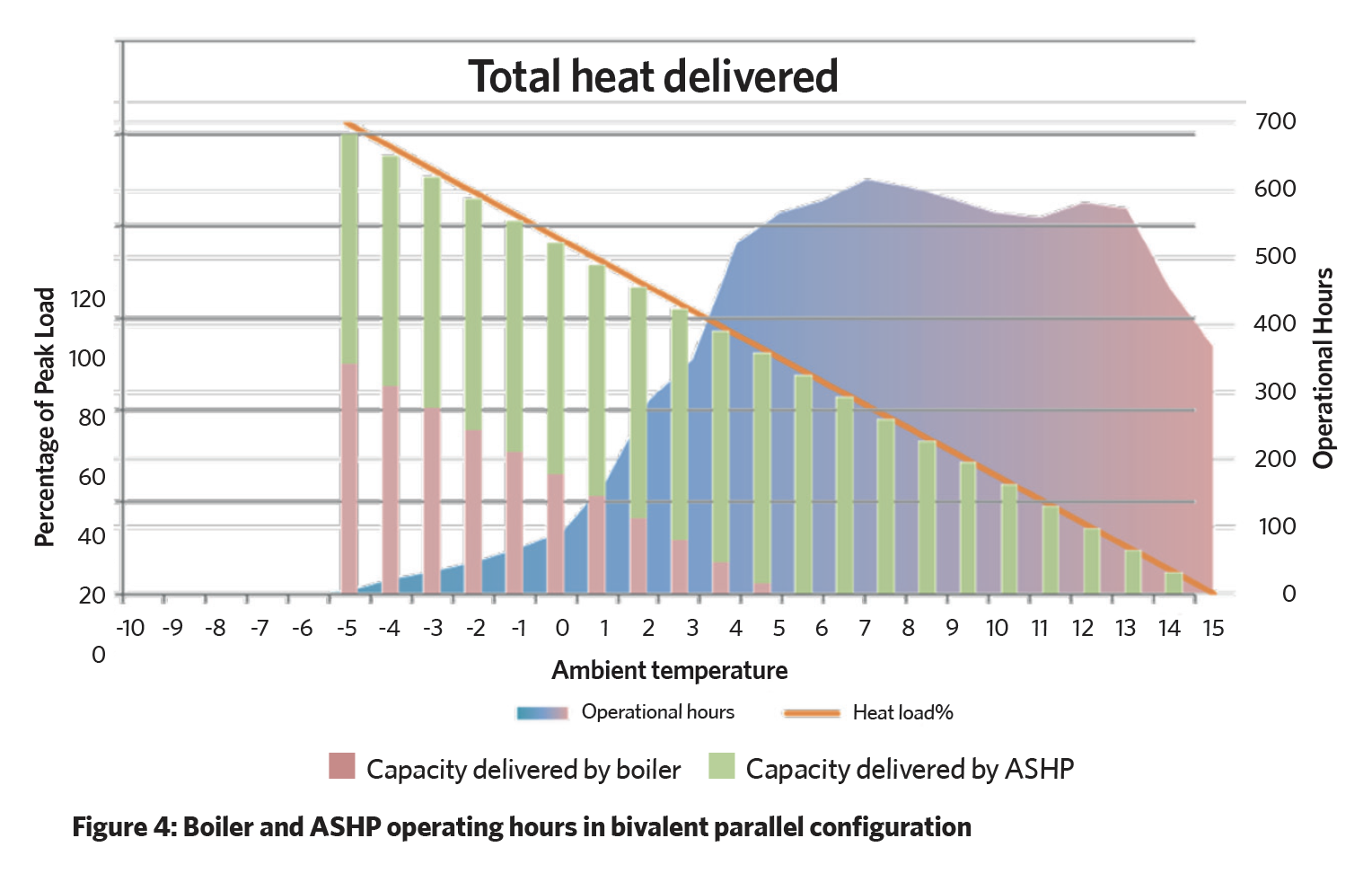
Special consideration must also be given to the choice of heat pump, to ensure it delivers the necessary flow temperature and capacity in all operational conditions. A cascade arrangement or high-temperature natural refrigerant product may be needed to achieve the required flow temperature. The capacity of the heat pump can be freely selected to meet any site constraints of power supply or plant space, and consideration can be given to capital costs to achieve the optimum balance.
To maximise the ratio of kWh contribution from the capacity of heat pump provided, our research shows the optimum capacity in relation to the building peak load is likely to be approximately 25-50%. See Table 2 for an example of a bivalent parallel arrangement. Calculations shown are for comparison purposes only
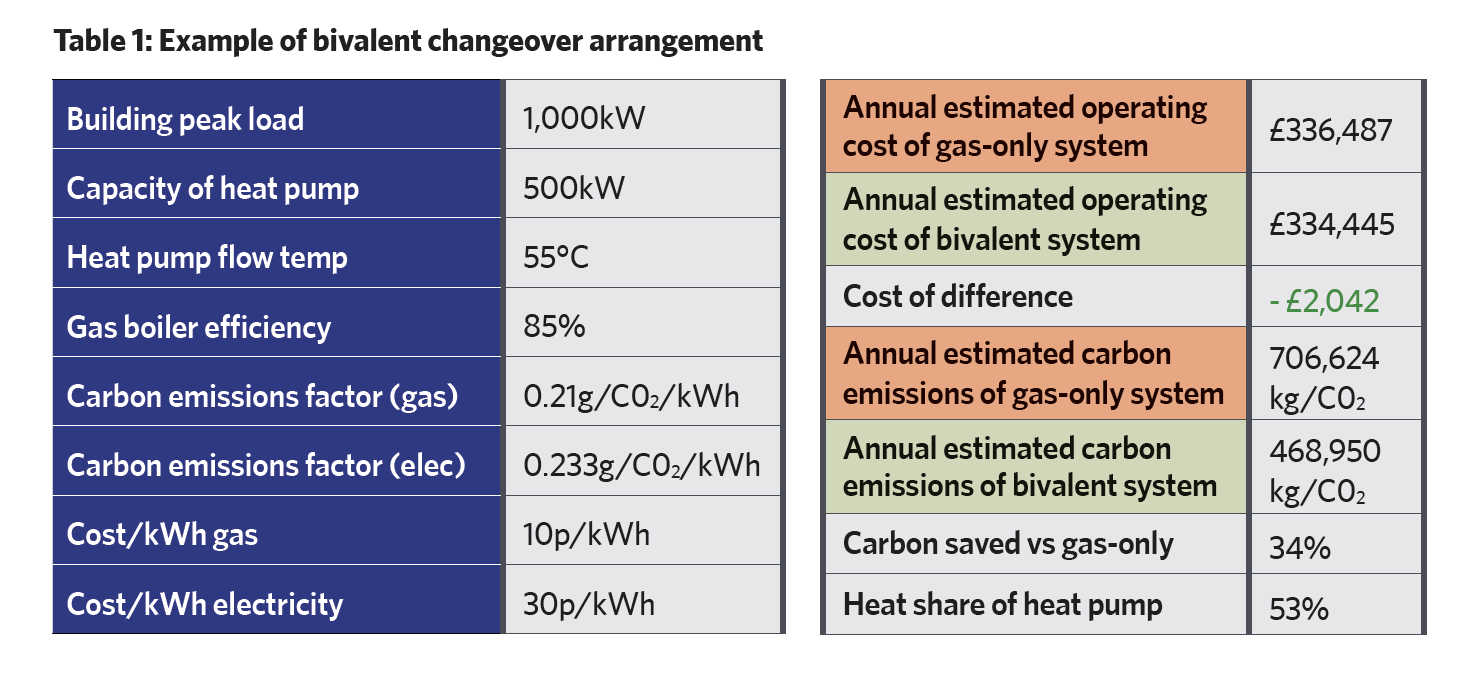
Conclusion
As the two examples show, introducing even a relatively small heat pump into an existing heating system will lead to carbon reductions, but applying that same heat pump capacity in different ways can achieve different outcomes.
Choosing which configuration and capacity of bivalent system is best suited for a specific project depends on budget, existing infrastructure, desired outcome and, most importantly, how it is controlled.