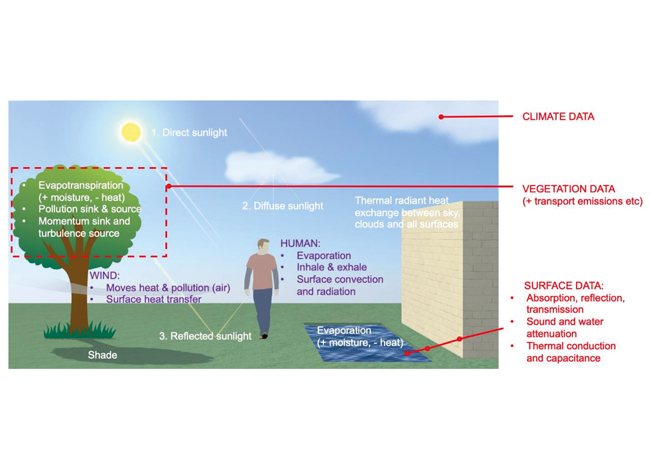
Figure 1: Urban physics – the science and engineering associated with air, heat, light, sound, water and surfaces
Cities are critical for solving problems associated with global climate change. They currently cover less than 1% of global land-surface area1, but account for 70-80% of CO2 emissions associated with energy consumption2.
As well as being a primary driver of climate change, cities are exposed to its negative consequences, and will have to adapt to extreme weather events, such as heatwaves and flooding. The vulnerable will be hit the hardest if society does not adapt to the changing climate.
One of the greatest environmental risks to our health is ambient (outdoor) air pollution, which leads to increased levels of heart disease, lung cancer and premature deaths. Almost everyone lives in environments exceeding World Health Organization (WHO) guidelines3, and some of our children are exposed to pollution levels that lead to reduced lung capacity and lifelong breathing disorders4.
Air quality, and its effects, may also be affected by a changing climate. It’s imperative that all routes to improving air quality should form part of a wider urban adaptation strategy.
In 2022, the UK Urban Environmental Quality (UKUEQ) partnership was established by the CIBSE Resilient Cities Group and UK Wind Engineering Society. Its aim was to help cities adapt to climate change by gaining a better insight into the urban environment – including air quality – through the use of urban physics and digital tools such as computational fluid dynamics. UKUEQ now consists of more than 20 organisations from industry and academia.
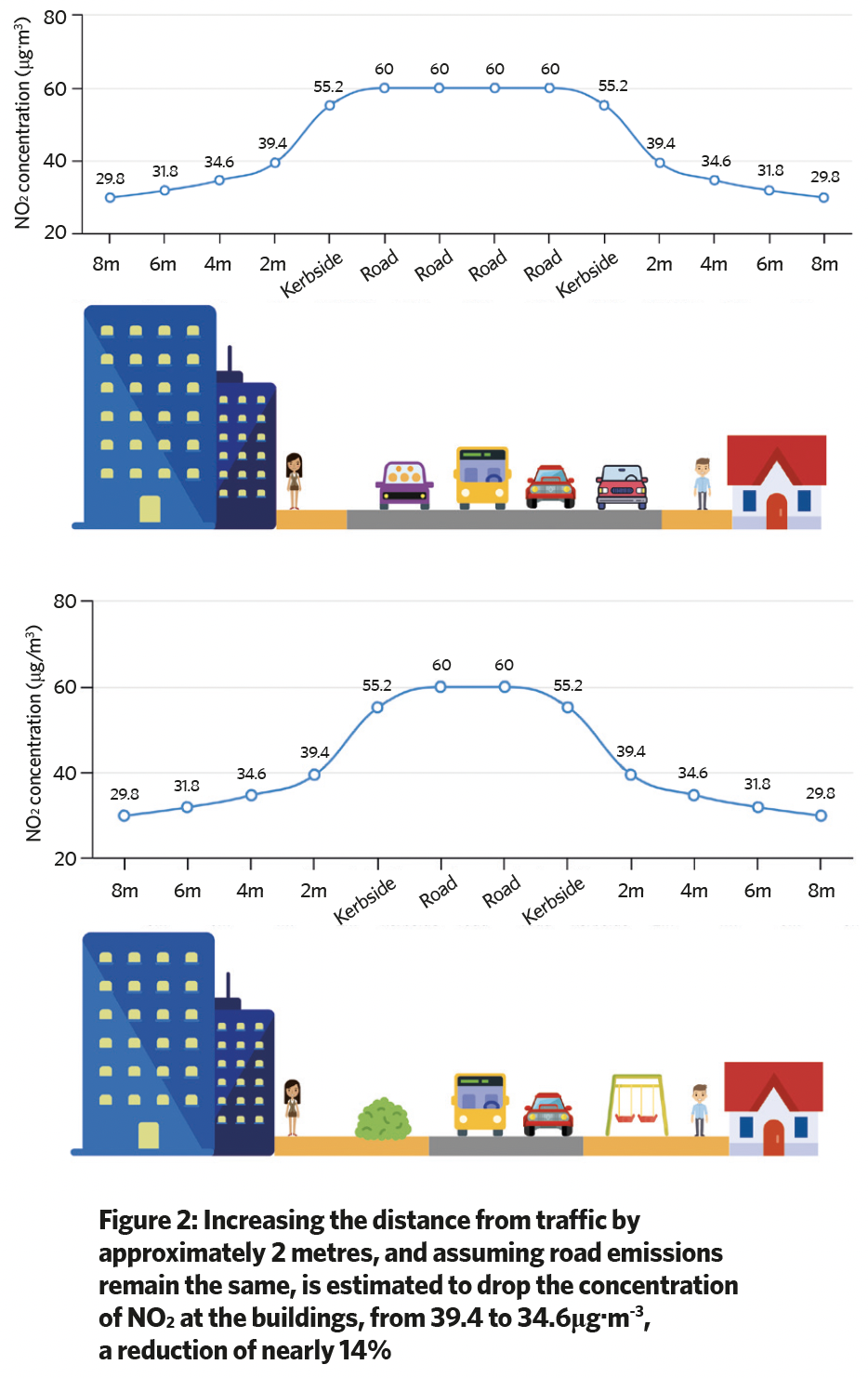
Some of the key factors determining the quality of the environment include wind, air quality, thermal conditions and acoustics (see panel). Urban physics is about understanding how the ingredients of this urban soup interrelate and are affected by other elements, such as light, water, surfaces, drainage systems and the urban form (morphology), which alters the natural exchanges of energy and water between the surface and air. Ultimately, the multiple factors influencing the urban environment drive the resulting conditions within designers’ models. (See Figure 1.)
For example, as air speeds near the surface increase, more surface heat is exchanged with the air through convection. Conversely, at lower air speeds, more surface heat is exchanged with the surrounding surfaces and sky through radiation. It is therefore important to take wind and urban morphology into account when looking to improve thermal and air quality environments.
Unfortunately, our industry’s design disciplines tend to work in isolation, with little communication at the engineering level. There is a need for increasing collaboration and integration to improve safety and performance, and future-proof our cities.
Early design discussions that consider urban physics can lead to significant gains for the environment. The impact of decisions should be assessed during the various design development stages.
Knowledge gaps remain significant, impeding all practitioners’ ability to enhance societal resilience and address poor environmental, energy and health issues. So, what is being done to fill knowledge gaps?
To increase resilience, more research and development is needed to improve methods, technology, and overall sustainability5. Currently, as a building industry, we still experience failures and poor performance when we engage with new technology.
Overall, there is much to do to bring all aspects into a collaborative and integrated approach to solve the problems of the future urban environment.
Air quality
Historically, problems with poor urban air quality were often driven by emissions from road transport and large industry. However, better regulation has led to large reductions in these emissions6. Poor urban air quality is now increasingly associated with the behaviour of individuals7, in the use of domestic wood burners, for example.
The most effective way to improve urban air quality is to reduce emissions at source, by avoiding certain activities or using alternative technologies. There are many ways this can be achieve during the construction and operation of projects8. Localised issues might be addressed by increasing the distance between pollution sources and sensitive users. This can be achieved with green infrastructure, to influence where people spend time and provide a barrier to the flow of air9. Plants and trees, and some commercial products, can remove pollutants from air, but are unlikely to achieve significant reductions on their own10,11.
Small changes to urban environments can result in large changes to pollutant concentrations, such as the reduction of space for motor cars leading to less traffic. Moving roads away from buildings can have significant benefits for building occupants (see Figure 2) and can lead to a modal shift away from polluting vehicles.
To reduce exposure to poor air, measures must be taken to improve both indoor and outdoor air, such as providing adequate ventilation, and minimising indoor emission sources and the ingress of outdoor pollutants12,13.
Wind
In cities where ‘day to day’ winds can be moderately high, such as in the UK, a new building can have a negative impact on the wind microclimate within the urban fabric. This is particularly relevant for buildings taller than their surroundings. Stronger winds impacting the upper portion of a high-rise can downdraught to street level and accelerate around building corners and between buildings.
Historically, pedestrian-level wind conditions in the vicinity of a new building development have been assessed, studied and mitigated using experimental wind-tunnel testing. More complex projects often require a series of wind-tunnel workshop sessions, where specialists guide the wider design team on the development of bespoke and site-specific aerodynamic solutions14.
In more recent years, to shorten the construction cycle time an ‘optioneering space’ has been generated, where data-driven approaches, machine learning-based assessments, and computational wind simulations play a much more important role in the feasibility and conceptual stages. This allows for a larger number of design options to be investigated, with wind-related risks better managed right at the start of the project, leaving confirmatory wind-tunnel tests for a later stage.
Thermal environment
Factors that play a vital role in defining the outdoor thermal comfort in an urban environment include: air temperature and relative humidity; mean radiant temperature; geometry and materials of buildings; and type of clothing and activity of users.
Guidelines published by the City of London Corporation15, together with new wind guidelines, aim to address thermal comfort and improve the quality of the public realm as part of the planning process. Bulk, shape and alignment of new developments can be impacted. The complexity and interplay of the different computational models required to assess urban microclimate conditions mean the process has yet to become common practice. The approach oversimplifies the effect of varying ambient surface temperatures by assuming mean radiant temperature is equal to air temperature. It can therefore only be considered a compliance, and not a performance-based, method.
Acoustics
The World Health Organization ranks noise pollution as the second most common urban environmental stressor in Europe15. Prolonged exposure to noise can lead to hypertension, annoyance, sleep disturbance, cognitive decline, and cardiovascular disorders. The annual social cost of urban traffic noise in England is estimated at £7-10bn16.
A recent research publication by the European Environment Agency estimated that 113 million people are thought to be annually impacted by long-term exposure to traffic noise levels of at least 55dB(A), day and night17.
However, the pursuit of an anti-noise quantitative approach may reduce sounds that are beneficial to our health and wellbeing. For example, the City of London Corporation18 recognises that noise resulting from the ‘buzz’ of urban areas is iconic, invigorating and an essential element of the city ‘soundscape’. It also recognises the need to provide respite from urban noise and encourages the identification and protection of relative tranquility.
- The UKUEQ is a working group of the CIBSE Resilient Cities Group. It will be hosting mini-symposia at the University of Birmingham and University of Southampton this year, and will again be hosting a seminar at CIBSE Build2Perform Live, taking place at London ExCeL from 13-14 November.
- For more details on UKUEQ and its activities and the white paper this article is based on please go to the Urban emergency article on the CIBSE website www.cibse.org/ukueq where you can access the full length version of this article
For the white paper this article is based on please go to the Urban emergency article on the CIBSE website
About the authors
Darren Woolf, chair of UKUEQ and the CIBSE Building Simulation Group, George Adams FCIBSE, CIBSE past president, and Stefano Cammelli, chair of the UK Wind Engineering Society
The authors would like to thank Ben Marner, at Air Quality Consultants, and Blaise Kelly, at TNO, for their contributions to the air quality sections.
References:
- Zhao et al (2022). A global dataset of annual urban extents (1992–2020) from harmonized nighttime lights, Earth Syst. Sci. Data, 14, 517–534. DOI:10.5194/essd- 14-517-2022
- Luqman et al (2023). On the impact of urbanisation on CO2 emissions. Urban Sustain 3, 6. DOI:10.1038/s42949-023-00084-2
- WHO (2022). Ambient (outdoor) air pollution fact sheet.
- Kings College London (2018). Air pollution restricting children’s lung development. https://www.kcl.ac.uk/news/air-pollution-restricting-childrens-lung-development
- Alexander Clifford (2023). Understanding the role of R&D in the construction industry.
- DEFRA (2016). NO2 fall off with distance calculator.
- DHSC (2020). Chief Medical Officer’s annual report 2022: air pollution.
- DEFRA (2022). Report: Non-methane Volatile Organic Compounds in the UK.
- Mayor of London (2023). Air quality positive (AQP) guidance.
- Mayor of London (2019). Using green infrastructure to protect people from air pollution.
- DEFRA (2018). Report: Impacts of vegetation on urban air pollution.
- DEFRA (2016). Report: Paints and surfaces for the removal of nitrogen oxides.
- DEFRA (2022). Report: Indoor air quality.
- Cammelli and Stanfield (2017). Meeting the challenges of planning policy for wind microclimate of high-rise developments in London. Procedia Engineering, Volume 198, 43–51.
- City of London Corporation (2020). Thermal comfort guidelines for developments in the City of London.
- Woolf, 2024. Cooking up an urban soup that tastes better. CIBSE Home Counties North West & Anglia Ruskin University seminar ‘Accounting for the urban heat island – Integrating multi-disciplinary perspectives for climate-responsive urbanism’, 18th January.
- World Health Organization (2018). Environmental noise guidelines for the European region
- Radicchi et al (2020). Sound and the healthy city. Cities & Health, 5:1-2, 1-13. DOI: 10.1080/23748834.2020.1821980