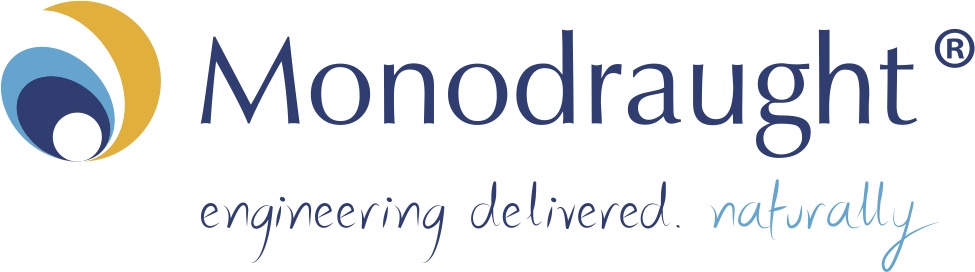
Designing for comfort
Different people can be thermally comfortable at very different temperatures because of their individual thermal histories and expectations. Comfort is influenced by personal and cultural assumptions – plus a cocktail of other variables – so it is impossible to predict precisely at what temperatures individuals will be comfortable.
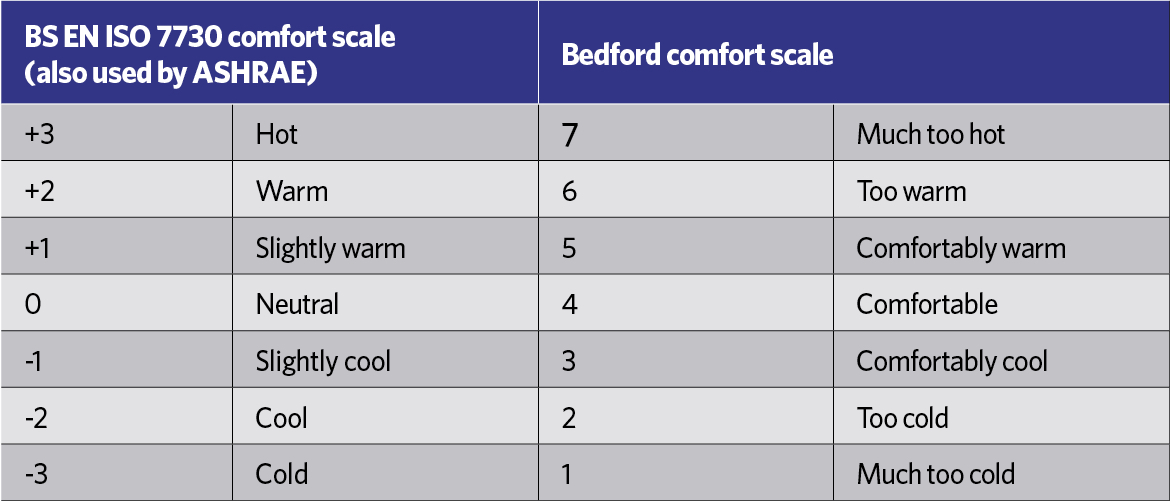
Table 1: BE EN ISO 7730 and Bedford comfort descriptors. The idea of ‘comfort’ is absent from the 7730 scale: people voting between –1 and +1 on this scale are generally assumed to be comfortable, and those voting +2 or +3 to be uncomfortably hot (Source: developed from CIBSE TM52, Table 1)
In recent years, the quest for sustainability has become more prominent, with different and potentially conflicting factors introduced. Fabric improvements – including more insulation and improved building airtightness – have been adopted, alongside technological developments that have resulted in the growing use of combined heat and power (CHP), heat pumps and heat-recovery systems. Together with the greater focus on ‘environmental ratings’, this technological evolution could be thought of as potentially deflecting attention from the consideration of a building’s ability to perform adequately in meeting the temporal variations in external temperatures and solar gains. As a consequence, the problems of overheating in inappropriately conceived buildings have led to the growing use of fans and mechanical cooling systems – with a consequent impact on energy consumption.
The quest for a robust and usable standard for comfort has been documented at least since David Boswell Reid experimented in the early 1800s1 and, latterly, with Thomas Bedford’s seminal work in the mid-1900s. The vocabulary for ‘comfort temperature’ morphs with each scrutiny, and ‘operative temperature’ is currently the accepted term, for which there are various abbreviated symbols (including Tcomf, tc, to, θO and θc). CIBSE uses θc.
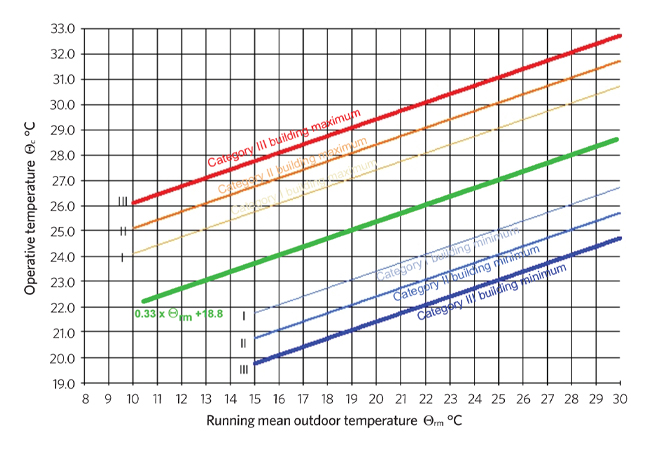
Figure 1: Acceptable operative temperature ranges for free-running, naturally conditioned spaces (Source: developed from BS EN 152514
There are two principal approaches used by designers to establish what constitutes a suitable environment in buildings and how to establish appropriate comfort temperatures – the ‘heat-balance model’ and the ‘adaptive thermal-comfort model’.
Heat-balance model
Developed in the last century, this model has traditionally been used by systems designers and modellers for buildings that rely on comfort being delivered through predominantly mechanical systems. Heat-balance models rely on a knowledge of the heat flows between the body and the environment.
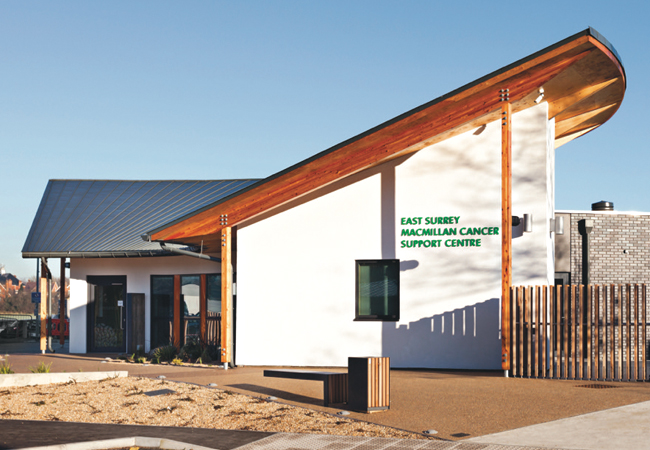
Figure 2: Shading reduces solar gain in this thermally slow response building and windtowers provide an unobtrusive method to enhance natural ventilation ©Michael Franke
The key is that, thermally, to maintain an acceptable heat balance for a particular person requires heat transfer by convection, conduction and radiation, and so will be determined by the air dry-bulb temperature, surrounding surface temperatures and air movement. It is not possible to predict these variables accurately, as they are contextually dependent not only on the occupants, their activities and acclimatisation, but also on the physical context of the modelled system – such as reflected solar gain, velocity of air, exposure of the site and variability of external climate as the weather changes. Particularly challenging in such calculations is the impact of potentially open windows.
The best known of heat-balance models is the predicted mean vote (PMV), developed by Ole Fanger.2 The PMV model is particularly important because it provides the basis for many commonly used national and international comfort standards. Its application can be inappropriate in naturally ventilated and mixed-mode buildings, and inflexible when used in conditioned buildings with multiple zones that are ‘thermally landscaped’ to save energy without affecting occupant comfort – for example, where significantly different controlled temperatures are used for corridors and toilets compared with non-transient areas.
Adaptive thermal-comfort model
People are actively engaged – and constantly working to keep themselves comfortable – in all buildings, whether they are naturally ventilated or air conditioned. People adapt to stay comfortable in a variety of ways:3
Physiologically: The body is constantly making subconscious adaptations to maintain its core body temperature of 37°C.
Behaviourally: This can be conscious, semi-conscious or subconscious. Examples include changing clothing, opening/closing windows, blinds or curtains, and using local heaters or fans.
Psychologically: This includes issues of expectations around the cost of energy, personality, status, ethical standards and control.
Adaptive comfort is measured in field surveys by asking people in buildings how comfortable they are on scales such as the BS EN ISO 7730 or Bedford – as illustrated in Table 1. The results of such surveys are then analysed statistically, to estimate the temperature at which the average survey participant will be comfortable. A useful elaboration on the application of comfort scales as survey tools can be found in BS EN ISO 10551 Ergonomics of the thermal environment. Assessment of the influence of the thermal environment using subjective judgement scales.
Recognition of the contribution and importance of this dynamic relationship between occupant and building underpins the adaptive comfort approach. The adaptive thermal-comfort model was initially developed to offer a more appropriate interpretation of the needs of low-energy and naturally ventilated buildings, and is recognised by CIBSE as being the appropriate method for undertaking such analysis.
Standards of comfort
These are the principal standards that are currently applied when considering comfort in buildings:
BS EN ISO 7730:2005 Moderate thermal environments – Determination of the PMV and PPD indices and specification of the conditions for thermal comfort
ISO 7730 sets out the heat balance comfort calculation and use of the predicted mean vote/predicted percentage of dissatisfied (PMV/PPD) index, as well as including some criteria for local comfort. The standard refers to ‘classes’ or categories of buildings according to the range of PMV that occurs within them. Category A buildings – with a notional PPD<6% – maintain their indoor environment within a very tight band of acceptable temperatures; practically, this could be regardless of whether a building is truly comfortable as determined by its particular occupants.
ASHRAE Standard 55:2013 Thermal Environmental Conditions for Human Occupancy
When ASHRAE 55 is applied to buildings with mechanical heating or cooling, it is similar to ISO 7730 in that it is based on PMV. The standard also defines acceptable thermal conditions for occupant-controlled, naturally conditioned spaces, where 80% to 90% of the building occupants might expect to find the conditions acceptable based on a comfort equation using a band of conditions centred on…
θc = 0.31·θpma + 17.8°C
θc is the nominal operative temperature (for comfort) and θpma is the prevailing mean outdoor air temperature (this is described more fully in section 5.4 of Standard 55, but is normally a ‘running mean’ temperature). For 80% of the occupants being satisfied, the limits are θc ± 3.5K, and to satisfy 90%, θc ± 2.5K.
BS EN 15251:2007 Indoor environmental input parameters for design and assessment of energy performance of buildings addressing indoor air quality, thermal environment, lighting and acoustics
BS EN 15251 uses categories for buildings (I, II and III) that are defined by the nature of the building rather than referring directly to the quality of its indoor environment. The adaptive standard defines acceptable values of the indoor operative temperature according to deviation from an operative temperature, θc, defined by the equation…
θc = 0.33·θrm + 18.8°C
where θrm is the exponentially weighted running mean of the outdoor temperature, which would normally be representative of at least the previous 10 days.
CIBSE recommends that ‘free-running’ buildings – those without mechanical heating or cooling – should conform to Category II (as shown in Figure 1), which sets a maximum acceptable temperature of 3K above the nominal comfort temperature. In summer, for most ordinary, free-running buildings, people can be considered to be comfortable indoors within a fairly wide temperature band over a day – varying by up to 8K – with the opportunity to open windows to facilitate year-round natural cooling. The very action of increasing the air speed from 0.1 to 0.6m·s-1 would increase the operative temperature for comfort5 by 2K.
Naturally conditioned buildings to meet the demands of occupant comfort
When using natural systems, indoor temperatures may fluctuate considerably over a day and across the seasons, but numerous studies have shown that occupants who are given local control are satisfied with a far wider range of comfort temperatures if they can negotiate, implement and accept conditions themselves.
The three main drivers for the climatic design of an individual building on a particular site are the wind, sun and rain. It is important that the whole design team considers the influence of the building shape and orientation by early review of sun paths and wind patterns, to maximise useful energy across the seasons, while avoiding overheating and excessive energy use. Buildings that are characterised as having a ‘slow’ thermal response are more able to maintain an internal environment where the temperature will not change too rapidly over the course of a day. (See CIBSE Guide A Section 5.6.4 for an explanation of thermal response.)
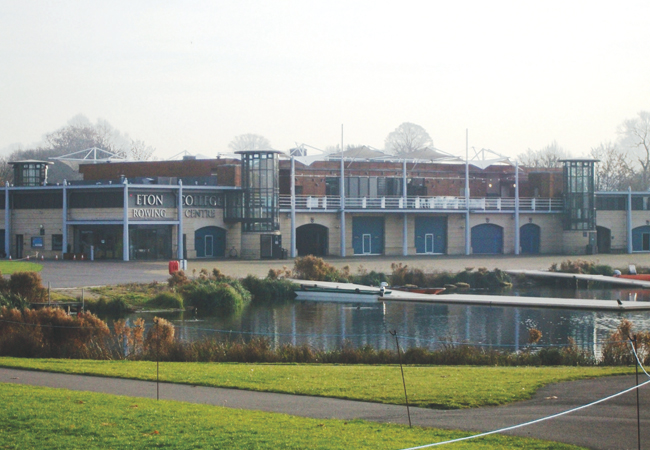
Figure 3: The Eton College boathouse incorporates four windtowers with integrated solar-powered fans. The natural ventilation brings a steady supply of outdoor air into the conference area, so maintaining good levels of IAQ (Source: Monodraught)
Materials that store heat accessibly in the building fabric can time-shift the energy flow so that it can be used internally when needed, rather than removing it from the building. Typically, buildings – such as that shown in Figure 2 – will benefit from being oriented towards the midday sun with appropriate structural shading and without excess glazing, and be constructed so as to allow for dumping excess heat via single-sided, cross or stack ventilation.
By understanding and using microclimate data in and around the construction, the building can be ‘landscaped’ into different temperature zones. In buildings such as the Eton Boathouse (Figure 3), thermal landscaping should take account of where ‘pools’ of heat or coolth existing around a building can be harvested, when necessary, to enhance the comfort of people indoors. For example, in conference centres, natural ventilation can be used to circulate fresh air around seated occupants to improve the concentration and focus of delegates, with optimum CO2 levels maintained. However, the sourcing of that airflow is also thermally critical.
Buffer spaces should be designed to protect against extreme thermal exposure, so giving an opportunity to decouple outside and inside temperatures.
Even if the building is already in operation, in a warming climate it is inevitable that more work will have to be done to maintain acceptable indoor conditions. Occupants should be encouraged – and educated – to take simple actions themselves and with their buildings to achieve more comfort. This can be as straightforward as adjusting clothing, opening windows, operating shutters, controlling shades and enabling people to move around spaces. In many cases, local mechanical systems can be applied ad hoc, to reduce peaks in heat/cold-stress.
The field of comfort is now recognised as a foundation stone in the design of low-carbon buildings, and fundamental for the enhancement of wellbeing, productivity and tolerance of occupants. An understanding of the principles of adaptive comfort is essential in designing and realising such buildings.
© Tim Dwyer, 2017.
- This article was developed from a paper by Professor Sue Roaf, director at Low Carbon Cities Initiative.
Further reading:
- Sections 1.3 and 1.4 of CIBSE Guide A 2015.
- CIBSE’s TM52, The limits of thermal comfort: avoiding overheating in European buildings.
- CIBSE Knowledge Series: KS16 How to manage overheating in buildings – A practical guide to improving summertime comfort in buildings.
- Short, A, The Recovery of Natural Environments in Architecture: Air, Comfort and Climate, Routledge 2017.
- Usable Buildings
- References:
- Schoenefeldt, H, Question time: Boswell Reid’s pioneering work on occupant evaluation in the Houses of Parliament, CIBSE Journal, September 2016.
- Fanger, P O, Thermal Comfort, Danish Technical Press, 1970.
- Humphreys, M et al, Adaptive Thermal Comfort: Foundations and Analysis, Routledge 2016.
- BS EN 15251:2007, Indoor environmental input parameters for design and assessment of energy performance of buildings addressing indoor air quality, thermal environment, lighting and acoustics, appendix A2.
- CIBSE Guide A, 2015, Section 1.3.1.1