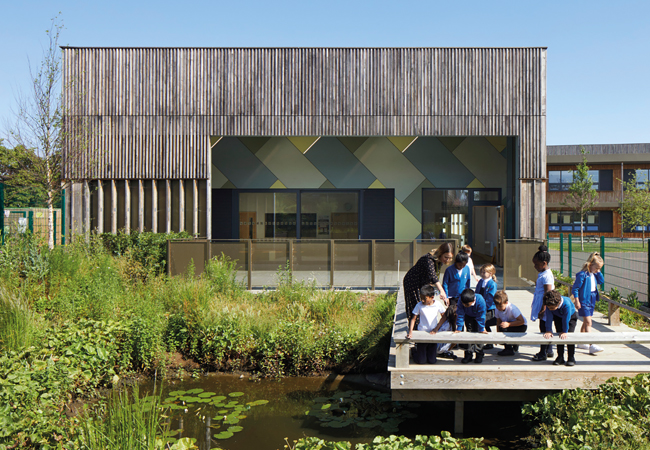
The Passivhaus Plus-certifi ed school has an all-timber superstructure
Hackbridge Primary is the first school building in the UK – and, potentially, the world – to be certified to the Passivhaus Plus standard. Completed in October 2019, this pioneering project in the London Borough of Sutton combines rigorous Passivhaus levels of fabric energy efficiency with renewable technologies, to deliver a school that generates more energy than it consumes over the course of a year.
This groundbreaking scheme sets a new benchmark for zero carbon educational buildings – an achievement acknowledged at the 2024 CIBSE Building Performance Awards, where it won Project of the Year – Public Use. The judges said the design team met the brief with an exceptional design that successfully combined ‘high-performing building fabric and high-performing engineering services’.
Project team
Client: London Borough of Sutton
Architect and Passivhaus consultant: Architype
M&E design: Introba (formerly Elementa)
Contractor: Willmott Dixon
Certifier: Warm
The school’s exemplary eco-credentials responded to the client’s ambitious ‘One Planet Living’ brief and were supported by planning requirements. Its location, between a conservation wetlands area on Metropolitan Open Land and the pioneering BedZED eco-village, meant that the school had to be zero carbon in operation, effectively, to gain planning approval.
Architype, the scheme’s architect and Passivhaus designer, suggested the Passivhaus Plus standard. ‘In 2014, we said, if you want to get this over the line with the planners you need to say you’re net zero, and the only way we believe you can demonstrate that is through Passivhaus Plus,’ says Christian Dimbleby, an associate at Architype, which worked with engineer Introba to develop the scheme.
The school stands on a shallow, insulated, concrete-raft foundation, incorporating ground granulated blast-furnace slag – a by-product of iron production – as a binder to lower the slab’s embodied carbon.
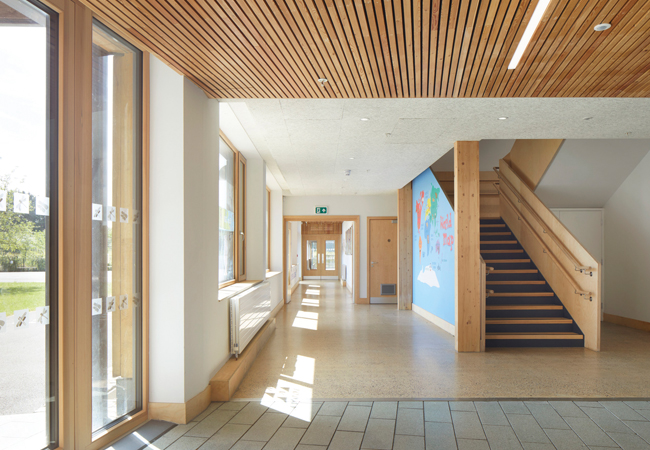
Its foundation is the only major non-timber element in the two-storey school’s construction. The entire superstructure, walls, roof, composite window frames, first-floor slab and cladding are all formed from timber, after Architype used ECCOlab software (an energy, carbon and cost calculator) early in the design process to select materials with low embodied carbon.
One of the benefits of Passivhaus levels of fabric insulation is that we didn’t have to locate the radiators under the window
To further reduce emissions, all soil excavated during construction has remained on site and has been used to create grass mounds that surround the playgrounds.
To minimise future embodied carbon emissions, partitions between rooms are designed to be moveable, to allow spaces to be easily reconfigured as the school evolves. Similarly, the school is designed to be extended easily to accommodate an increased pupil intake, without the need for reconfiguring the existing structure or major interventions on the building services.
‘We’ve designed the building so the circulation spaces, assembly hall and plant work for a two-form entry,’ says Dimbleby.
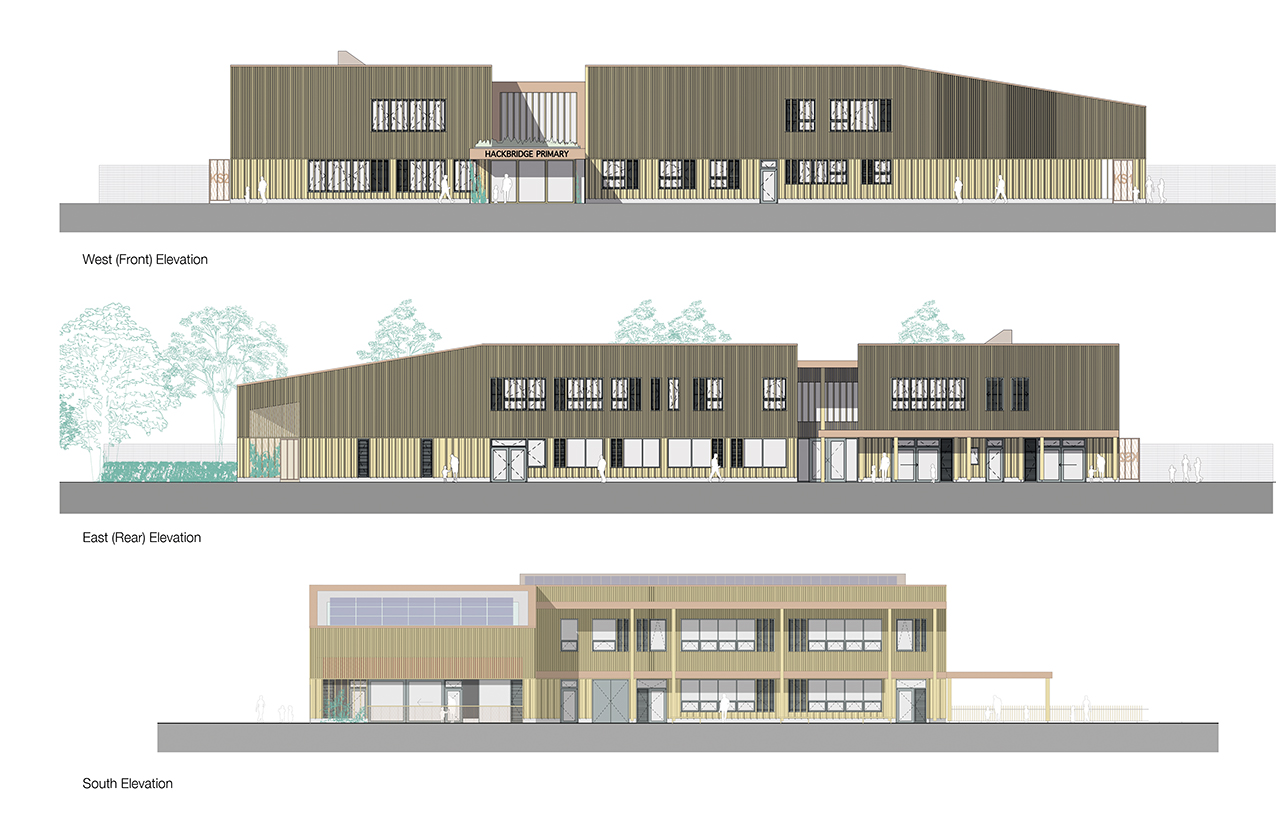
The west, east and south elevations of Hackbridge Primary School
As a Passivhaus building, the L-shaped school has a highly insulated and airtight envelope, to minimise fabric heat losses, control heat gains and provide excellent levels of comfort. The large school hall is positioned facing east-west and serves to help block traffic noise from the nearby main road. The hall shelters the adjoining classroom wing, which faces north-south to optimise solar gains.
Brise soleil and an oversailing roof provide shade to the southern elevation to prevent the classrooms from getting too warm in summer, in compliance with Passivhaus overheating criteria and CIBSE TM52.
‘We did a lot of work with Introba on optimising window positioning and sizing,’ Dimbleby explains.
Classrooms have mixed-mode ventilation, allowing teachers to open windows when conditions are suitable.
Classrooms are provided with mixed-mode ventilation. When conditions are suitable, teachers can open the windows. For the remainder of the time, an AHU with thermal-wheel heat recovery ducts fresh air to a bulkhead at the back of the classrooms. Air returns through acoustic attenuated transfer ducts into the circulation corridors, from where it can permeate into the school hall and the toilets and be ducted back to the AHU, so that heat or coolth can be recovered before the stale air is discharged. In addition, when the kitchen is in use its extract incorporates a runaround coil to capture heat from the exhaust air, which is also used to temper the supply air. This cascade approach to ventilation means there is no need for an additional air supply to the main hall because, if pupils are in there, they are not in the classrooms, so the fresh air they would have consumed will make its way through the building’s core to the hall. ‘The concept was accepted by Building Control, which enabled us to tick the Passivhaus ventilation criteria,’ explains Simon Ebbatson, senior principal at Introba. Budget constraints prohibited a variable air volume system, so the ventilation is constant volume, controlled on a time clock. The controls do, however, incorporate a reduced-volume mode for community use of the hall at evenings and weekends. In this mode, fresh air is not supplied to the classrooms but directly to the hall and admin areas used by the community.Ventilation strategy
In winter, heating is provided by an ICAX 70kW ground source heat pump (GSHP) system. This extracts heat from the ground via eight, 130m-deep boreholes and stores it at 42oC in a 300-litre thermal store.
A low-temperature hot water circuit, operating at 42oC flow/37oC return, supplies low-temperature radiators in the classrooms and an oversized heating coil in the air handling unit (AHU).
‘One of the benefits of Passivhaus levels of fabric insulation is that we didn’t have to locate the radiators under the window,’ says Graham Day, associate principal engineer at Introba.
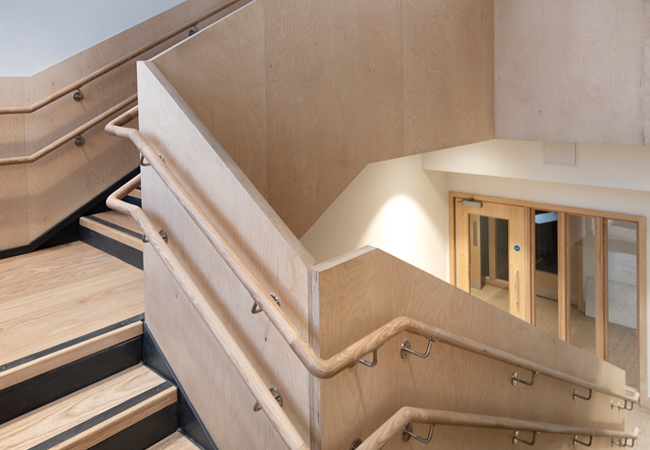
The school meets LETI embodied carbon targets
Using the ground as an interseasonal heat store enables the boreholes to provide cool water to temper the fresh air supply in summer. In this mode, the heat pump is bypassed so that ground-temperature water (at about 10oC) is circulated to the AHU cooling coil. Coolth is also recovered from exhaust air by the AHU’s thermal wheel.
‘While we’re extracting coolth from the ground we’re warming it up, which helps the heat pump operate more efficiently in winter,’ Day explains. Because supplying cooling only requires a very small amount of power to run the circulating pumps, it is beneficial to do so, adds Day, because it enables the heat pumps to work more efficiently in winter. ‘It is better than free cooling – it is beneficial cooling,’ he says.
For optimum system performance, annual heating and cooling loads should be balanced, so the heat removed from the ground in winter is put back in summer. ‘On this scheme, we’ve not managed to achieve a precise balance, but there is still a beneficial heat exchange,’ Day says.
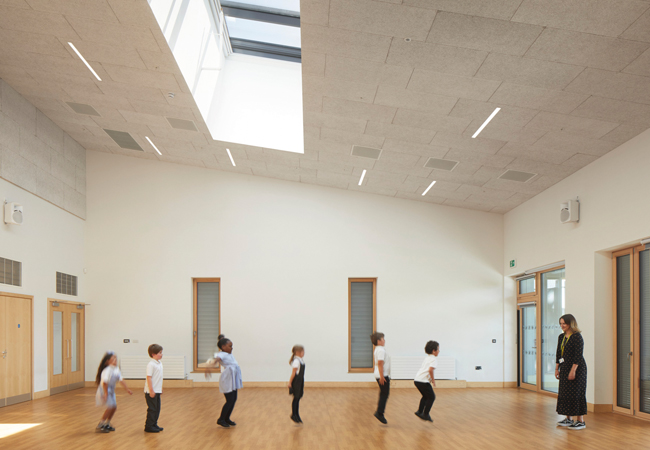
Opening windows are key to the Passivhaus strategy
A separate 40kW heat pump connected to the same borehole array is used to preheat the domestic hot-water thermal store to 52oC. A gas-fired water heater is used to provide top-up heat to the vessel and is available to provide backup to the heating system. ‘Improvements in technology mean that, if we were designing the system today, we’d be able to heat the domestic hot water entirely by heat pump,’ explains Simon Ebbatson, senior principal at Introba.
The temperature in the domestic hot-water network is maintained using a conventional circulation system, but with microbore pipework connections to individual taps.
‘Passivhaus penalises you heavily on dead-leg lengths, so we’ve designed the system to ensure there is no more than a litre of water in any one of these,’ says Day.
To achieve Passivhaus Plus, the school must offset all energy use over the course of a year, including unregulated loads. To do this, it incorporates 424m2 of photovoltaic (PV) panels, designed to deliver 81kWp. The panels are supported on frames above the green roof, where a symbiotic relationship means that panels provide shade to the vegetation, while transpiration from the vegetation and evaporation from the growing layer,help cool the panels, improving their output.
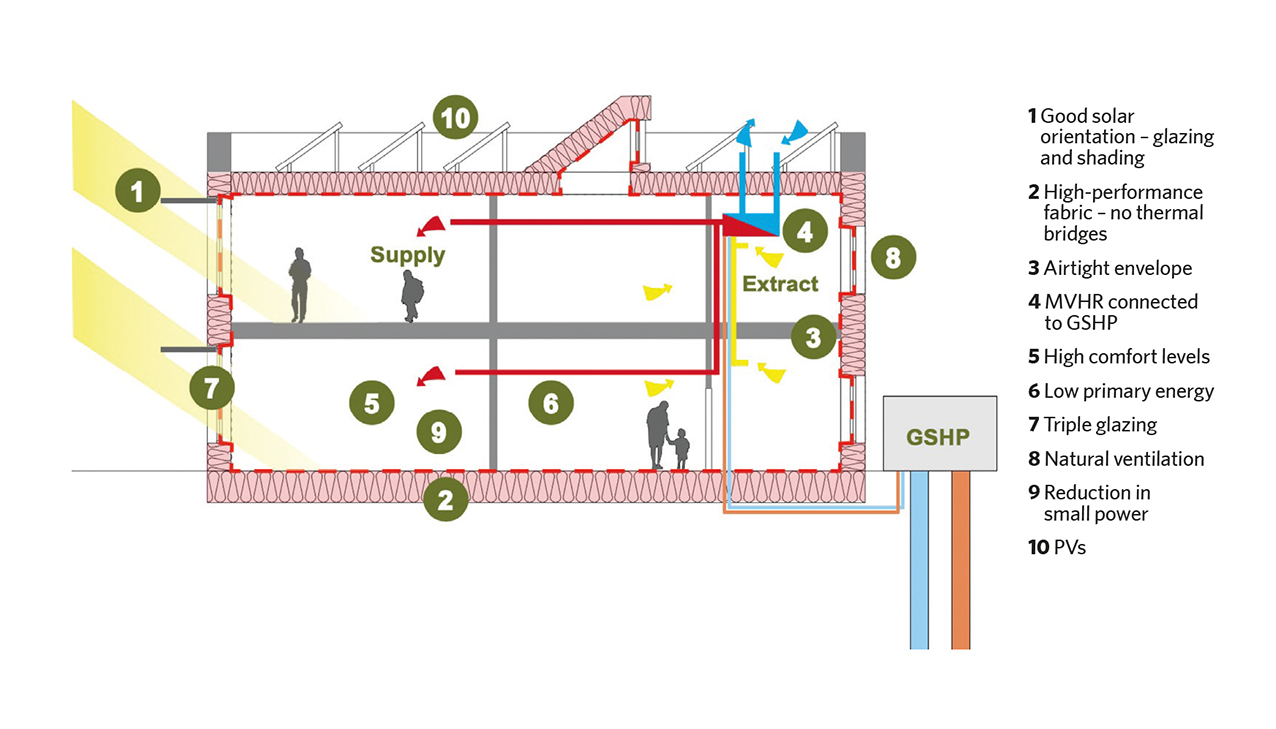
Figure 1: EUI of Hackbridge Primary School compared with CIBSE typical and good practice, and LETI school benchmark
The downside of using PVs to achieve an energy balance on a school is that the panels provide the highest electrical output on summer days, when the school is closed. While the school can sell this surplus electricity to the Grid at a relatively low price, it does still have to buy electricity from the Grid at a much higher price to make up for the electricity shortfall on shorter, darker winter days. The school achieves an annual energy balance, but there is still a net cost for electricity. ‘Unfortunately, achieving an energy balance does not necessarily result in a financial balance in today’s market; we hope this may change in future,’ Day says. See Figure 1 for energy performance data.
The latest sub-metered data shows energy consumption is very close to the PHPP design targets and on track to achieve net zero energy
The school received its first intake of pupils just before the Covid pandemic, which hindered its operational optimisation and, subsequently, its ability to achieve net zero energy in operation. There were also issues with a faulty buffer vessel and three of the photovoltaic inverters failed. School staff, too, struggled to come to terms with the nuances of running a Passivhaus Plus school – for example, knowing when to open the windows and when to take advantage of free cooling.
To their credit, the engineers and architect have remained committed to the project and have continued to be involved through a voluntary soft landings arrangement. In addition, contractor Willmott Dixon has implemented its Energy Synergy process, to measure and verify the school’s operational energy use by comparing metered data against monthly Passive House Planning Package (PHPP) targets, which has helped highlight some anomalies. For example, a building management system (BMS) demand signal to the GSHP stopped working after a software update to the BMS. This was picked up by a sudden increase in gas consumption.
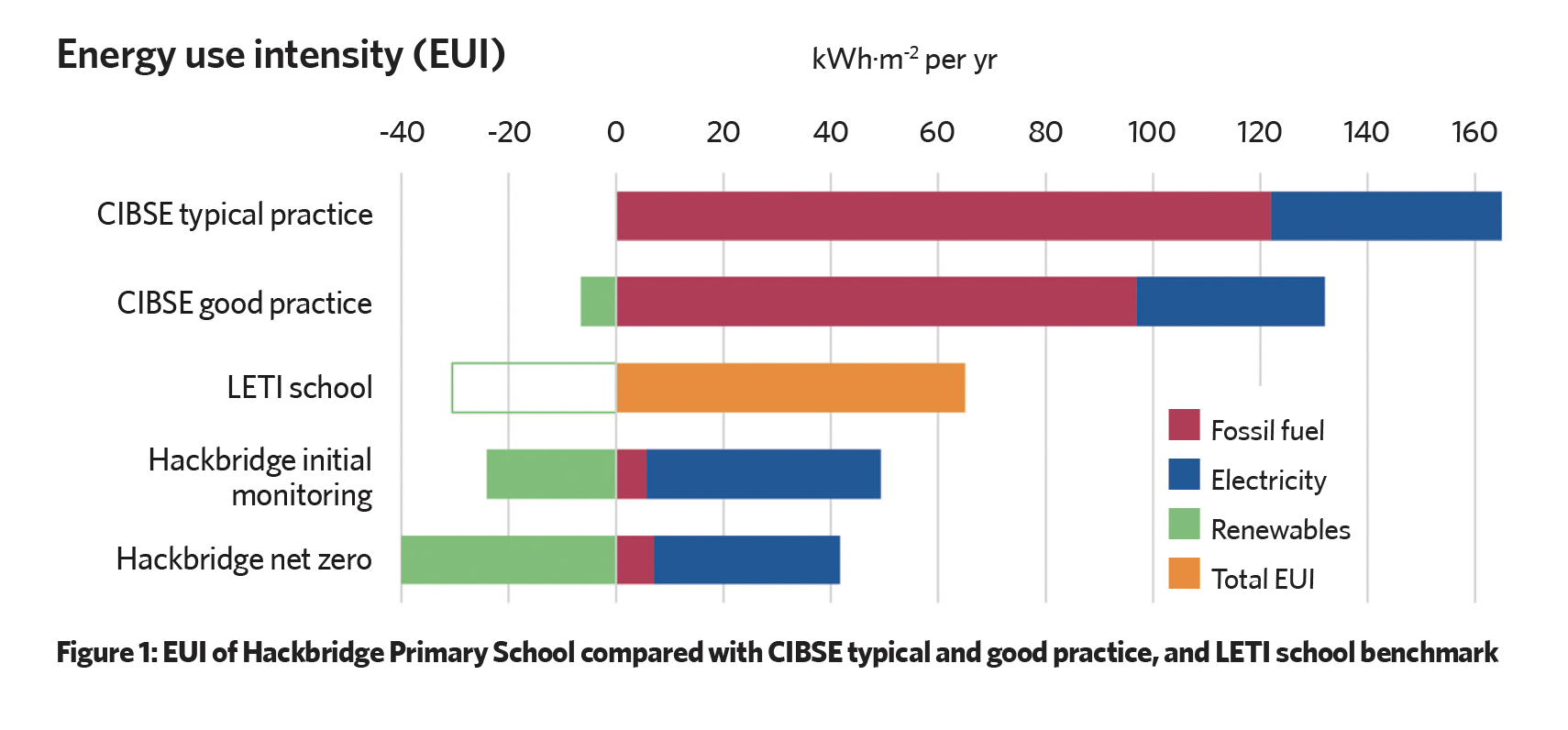
Energy
Operational energy
- Target annual energy use: 77,876 kWh per yr
- Actual metered energy use: 85,861 kWh per yr
- Total energy produced by PVs: 45,077 kWh per yr
Embodied carbon
- Target upfront embodied carbon: No target formalised <500kgCO2e/m2 A1-A5
- Actual as-built embodied carbon: 499kg/CO2e/m2 A1-A5 (excluding sequestration), 405kg/CO2e/m2 A1-A5 (including sequestration)
The team’s perseverance has paid off; four years after the school opened, the latest sub-metered data shows energy consumption and generation is very close to the PHPP design targets and on track to achieve net zero energy (see Figure 1).
This pioneering school is finally living up to its A+ Energy Performance Certificate billing. With an energy use intensity of 42kWh.m-2 per yr, Architype says the school exceeds RIBA’s 2030 operational targets and, with upfront embodied carbon with sequestration of 405kg CO2e.m-2, meets LETI’s 2020 embodied carbon targets.
The building’s impressive green credentials are used to enrich the school curriculum and bolster environmental awareness among the pupils. This makes it a great example of how we can embed environmental design in our school buildings and inspire the next generation.