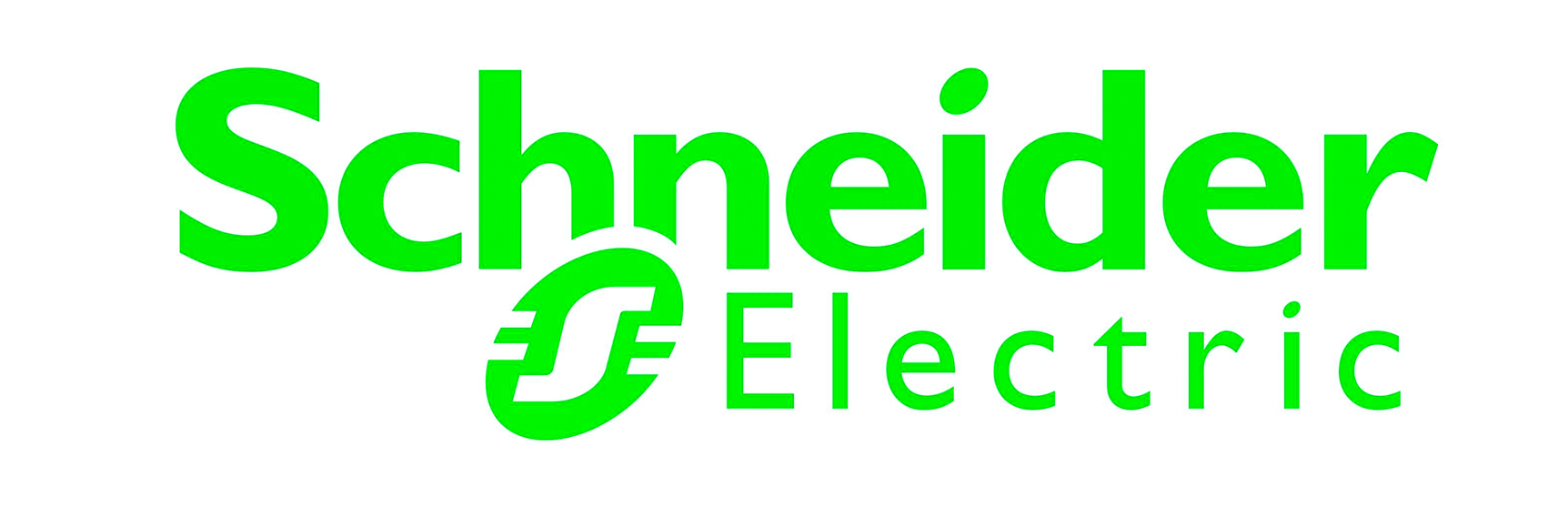
Historically, prior to the advent of solid-state electronics, alternating current (AC) power quality was defined primarily by voltage, frequency and power factor. If these aspects were maintained within industry tolerances, then it would have been reasonable to expect the consumers – domestic, commercial, institutional and industrial – to be suitably supplied with electrical power.
Since the advent of solid-state electronic equipment, however, there has been an increasing need to consider other more complex factors – such as harmonics, transients and momentary disruptions – all of which can result in:
1. Unexpected business downtime
- Power quality disturbances account for 30-40% of business downtime
- Poor power quality costs 4% of annual turnover (industry sector)
- Estimated financial loss of €150bn (£110) per year in Europe1
2. Equipment malfunction and damage
- Overheating of motors, capacitors, cables and transformers
- Accelerated wear and tear on critical components
- Improper function
- Premature ageing
- Nuisance tripping of circuit breakers
Some 70% of the power quality disturbances originate within the consumer’s premises, while 30% are on the network side.2
Some important changes and developments that have occurred in recent years also increase the need for assessment and maintenance of power quality:
- There is a greater dependence on continuous availability of electric power
- E-commerce is changing the way all spheres of human activity are interacting with the world
- Intelligent technology demands power that is free from interruption or disruptions
- Use of renewable energy has resulted in an increase in power quality issues
- The level of quality perceived by the supplier (or regulator) may be different from what the customer requires or desires.
Power quality phenomena and causes
Power quality issues should be considered in terms of the time and duration over which effects occur. Long-term power quality issues – such as power system harmonics, unbalanced phases, under and over voltages, frequency variations, voltage fluctuations (flicker) and poor power-factor – can lead to equipment failure, malfunctions, overheating and damage. Short timescale power quality issues – including short-duration voltage variations, interruptions and dropouts, sags and swells and system transients –may well result in visible and immediate impact, including reduced efficiency, potential malfunction and damage, all resulting in unscheduled downtime.
Standards and regulations
It is not only the effect that other systems might have on a particular installation, but also the effect that that installation has on others.
The Distribution Code3
This code covers technical aspects relating to the connection and use of the electricity distribution licensees’ distribution networks. It is a cross-industry code, developed by industry, including suppliers, and the UK government.
The district network operator (DNO), as well as generators, suppliers and customers connected to, or seeking connection to, the DNO’s distribution system must comply with the code. The DNO and all users have a duty under this Distribution Code to provide such information and resources as are necessary to facilitate compliance with, and implementation of, the Distribution Code. The Distribution Code contains guidelines for all aspects of the supply industry; of particular significance to the power quality is the Distribution Planning and Connection Code (DPC) that contains:
DPC4.2.2 Frequency and voltage – Requires that the voltage and frequency of the system complies with the Electrical Safety, Quality and Continuity Regulations.4
DPC4.2.3 Voltage disturbances and harmonic distortion – DPC 4 recognises that in fault conditions, the voltage may fall significantly at the moment of fault. These transient effects are heavily affected by system design and, in particular, the earthing system design.
Reference is made to BS EN 50160:2010 Voltage Characteristics of Electricity Supplied by Public Distribution Systems (discussed below).
DPC 4 also requires that the harmonic content of any connected load must comply with the limits set out within Annex 1, Item 1 Engineering Recommendation G5/4-1, Planning levels for harmonic voltage distortion and the connection of non-linear equipment to transmission and distribution systems in the United Kingdom.
BS EN 50160:2010 Voltage characteristics of electricity supplied by public electricity networks5
This standard describes and specifies the characteristics of the supply voltage that can be expected from the DNO, and covers frequency, magnitude, waveform and symmetry of the line voltages, defining acceptable levels, measurement periods and intervals of power quality phenomena, including:
- Power frequency
- Flicker
- Supply voltage dips
- Short and long interruptions
- Temporary over-voltage
- Supply voltage magnitude
- Supply voltage unbalance
- Harmonic voltage
- Inter-harmonic voltage
- Mains signalling voltage
Engineering recommendation G5/4-16
This standard was issued by the Energy Networks Association (ENA) and defines the permitted levels for harmonic voltage distortion and the connection of ‘non-linear’ equipment.
G5/4-1 aims to ensure that the levels of harmonic current in the public electricity supply system do not give rise to problems for connected users.
G5/4-1 provides the standard basis of assessment for use by network operating companies (NOCs) and their customers, which then forms part of the connection agreement signed by the NOC and customer.
Maximum permissible harmonic currents are defined within G5/4-1 and tabulated in Table 7 of the document.
To measure and assess harmonic content of systems properly, all measurement devices – including fixed meters – need to be IEC 61000-4-30 Class A-compliant.
Developing a strategy
The design of systems and means to mitigate export of power problems to the public supply network (and across a local network) is beyond the scope of this article.
However, even with the best designed system there is still a risk that an installation might either affect other systems, or that it might be affected by other systems.
A monitoring system may be found to be the most cost-effective and robust way to manage this risk, but there should be an overall strategy to monitor and manage power quality.
1) Determining goals for the monitoring system
a) Energy metering
Systems that record energy usage (kWh), voltage, current and power demands are widely applied in buildings. Measurements can be made at a site, building or even equipment level, and can be collected at regular time intervals (such as half-hourly). These results can inform operational analysis and have benefits for energy management, load reduction, peak levelling and billing.
CIBSE TM397 offers a useful methodology for this type of monitoring. Instantaneous and customised logging of parameters can also provide insight into performance and network health. Such monitoring is commonly managed by a building management system (BMS), or dedicated energy management system.
However, these systems often provide neither the measurements, nor the resolution, needed to monitor and report on power quality.
b) Power quality metering
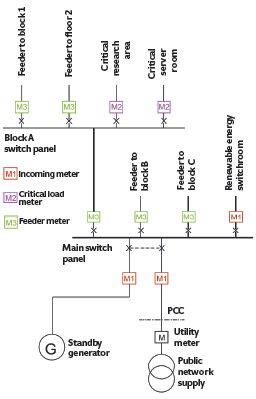
Figure 1: Typical simple distribution system
These systems use more specialist meters and often include a dedicated recording network. They are typically aimed at maximising the efficiency, safety and reliability of the electrical infrastructure, and measuring power quality, as well as being capable of:
- Maximising the efficiency and reliability of the electrical infrastructure
- Measuring the quality and quantity of power in the system
- Offering sufficient time-domain resolution
- Maximising system uptime
- Improving maintenance response
- Ensuring power quality compliance
- Network protection and control
2) Selecting and appropriately locating the correct types of meter to capture data and transient events
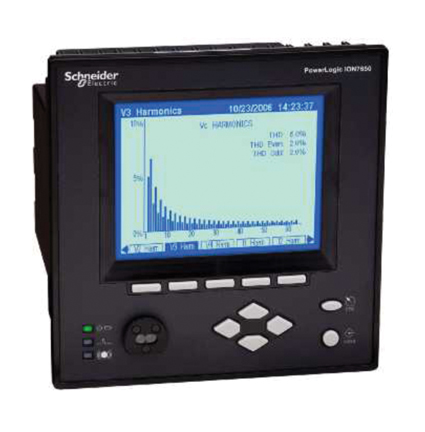
Figure 2: Power meters (incoming) High accuracy (0.2 class); very high sample rate (>512 per cycle); energy usage monitoring; load monitoring; reliable data; basic power quality monitoring (V, I, kW, kVA, PF and total harmonic distortion); advanced powerquality recording (sags, swells, outages, transients); utility bill verification (check accuracy of utility bills); alarm configuration (to capture events and time index them); additional inputs (gas, water and so on.); EN50160 reporting and IEC61000-4-30 Class A-compliant for harmonic measurement to G5/4
The hardware, firmware and software sophistication of a meter are generally related to its cost. It is, therefore, important to select the correct meter for application at a given point in the system. Complex meters provide greater accuracy and more information, but might not be needed at all points in a network (see Figure 1).
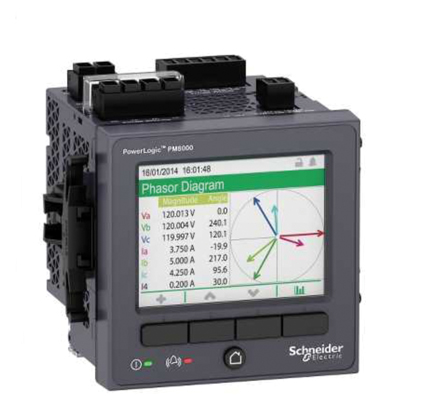
Power meters (critical loads) High accuracy (0.2 class); high sample rate (>256 per cycle); load monitoring; reliable data; basic power quality monitoring (V, I, kW, kVA, PF and total harmonic distortion); advanced power quality recording (sags, swells,outages, transients); alarm configuration (to capture events and time index them); additional inputs (gas, water and so on)
A key location to measure power quality is at the main switch panel. This will effectively provide data and information on the point of common coupling (PCC), as defined in G5/4-1, and hence enable assessment against this and other standards. Meters with a high degree of information and good resolution should be installed on incoming supplies, including all sources such as generators, renewable energy and dual supply points.
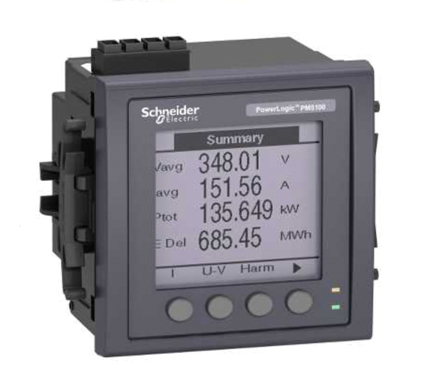
Power meters (feeder) Good accuracy (0.5 class); energy usage monitoring; load monitoring; reliable data; basic power quality monitoring (V, I, kW, kVA, PF and total harmonic distortion); additional inputs (gas, water and so on)
Outgoing supplies should also have meters fitted. It is sensible to provide high functionality meters for critical loads or main feeders that serve non-critical loads. Although small supplies may necessitate less complex meters, it is best to confirm what type of loads are envisaged, as that individual supply to plant or outbuilding might end up being a source of a problem. Typical applications of meters are shown in Figure 2.
3) Applying the most appropriate software to visualise results and provide analysis
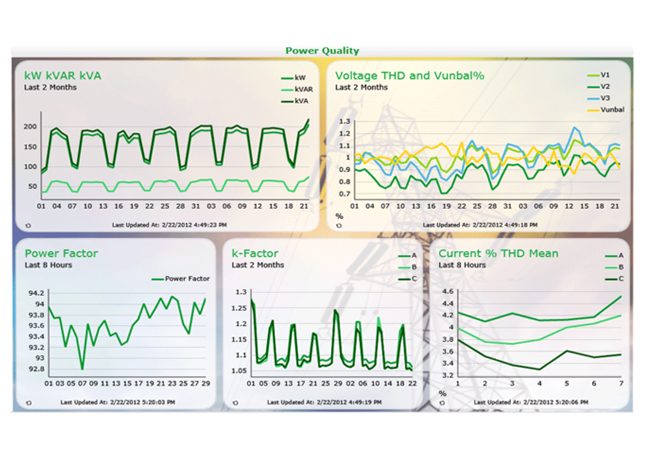
Figure 3: Graphical views
Meters that store data and capture events are available; more basic meters will provide real time readouts that must be collected and stored elsewhere. Monitoring software should be able to communicate with all types of meter used, and then be able to collate and present data so that the user can carry out analysis. Ideally, software should be able to: acquire data from multiple sources; have long term storage and data history; allow logical application of ’virtual metering‘; and allow data to be shared or exported to other packages.
The software is often able to present data in a visual form that enables easy but accurate assimilation of information and viewing of results.
4) Make informed decisions based on results
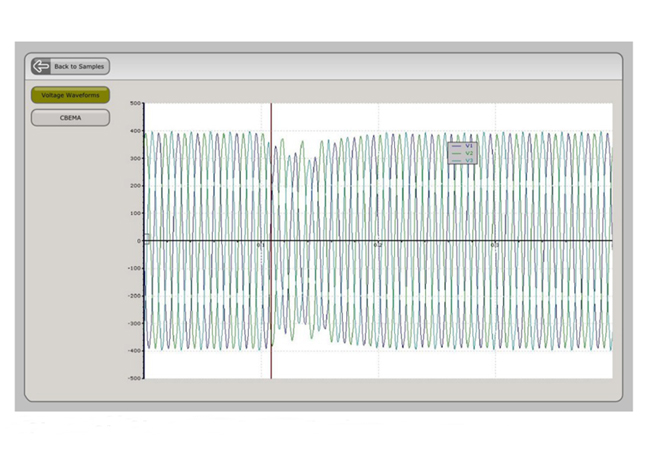
Figure 4:Short-duration voltage variance
Once power quality data is recorded and collected, the findings should be used to determine the health of the system and, if necessary, influence the design or investigation of options for mitigation measures or improvements.
Thereafter, monitoring and vigilance is necessary to maintain compliance and correct operation.
The ‘internet of things’, ‘smart cities’ and spreading use of embedded intelligence in all aspects of life means that power quality cannot be ignored and will become increasingly important in years to come. Awareness and a strategy to monitor and manage effects are essential.
© Adam Rawlinson, 2015.
Further reading
BSRIA Power Quality Application Guide AG2/2000. Copper Development Association – Electrical Design – A Good Practice Guide, 1997. Section 3.
References:
1 Manson J and Targosz R – European Power Quality Report, Leonardo Energy, November 2008, section 5, page 8.
2 Bhattacharyya S and Sjef Cobben S Consequences of Poor Power Quality – An Overview, Technical University of Eindhoven, from Power Quality, edited by Eberhard A, Intertech Web 2011. www.intechopen.com. Part 1, chapter 1, section 2.
3 Distribution Code of Licensed Distribution Operators of Great Britain, DPC 4. www.dcode.org.uk/the-distribution-code/
4 Electricity Supply Quality and Continuity Regulations 2002, HM Stationery Office. SI 2665
5 BS EN 50160:2010 + A1:2015, British Standards Institution.
6 Engineering Recommendation G5/4-1 Planning Levels for harmonic Voltage Distortion and the Connection of Non-Linear Equipment to Transmission Systems and Distribution Networks in the United Kingdom, Energy Networks Association, 2005.
7 CIBSE Technical Memorandum TM39 Building Energy Metering.