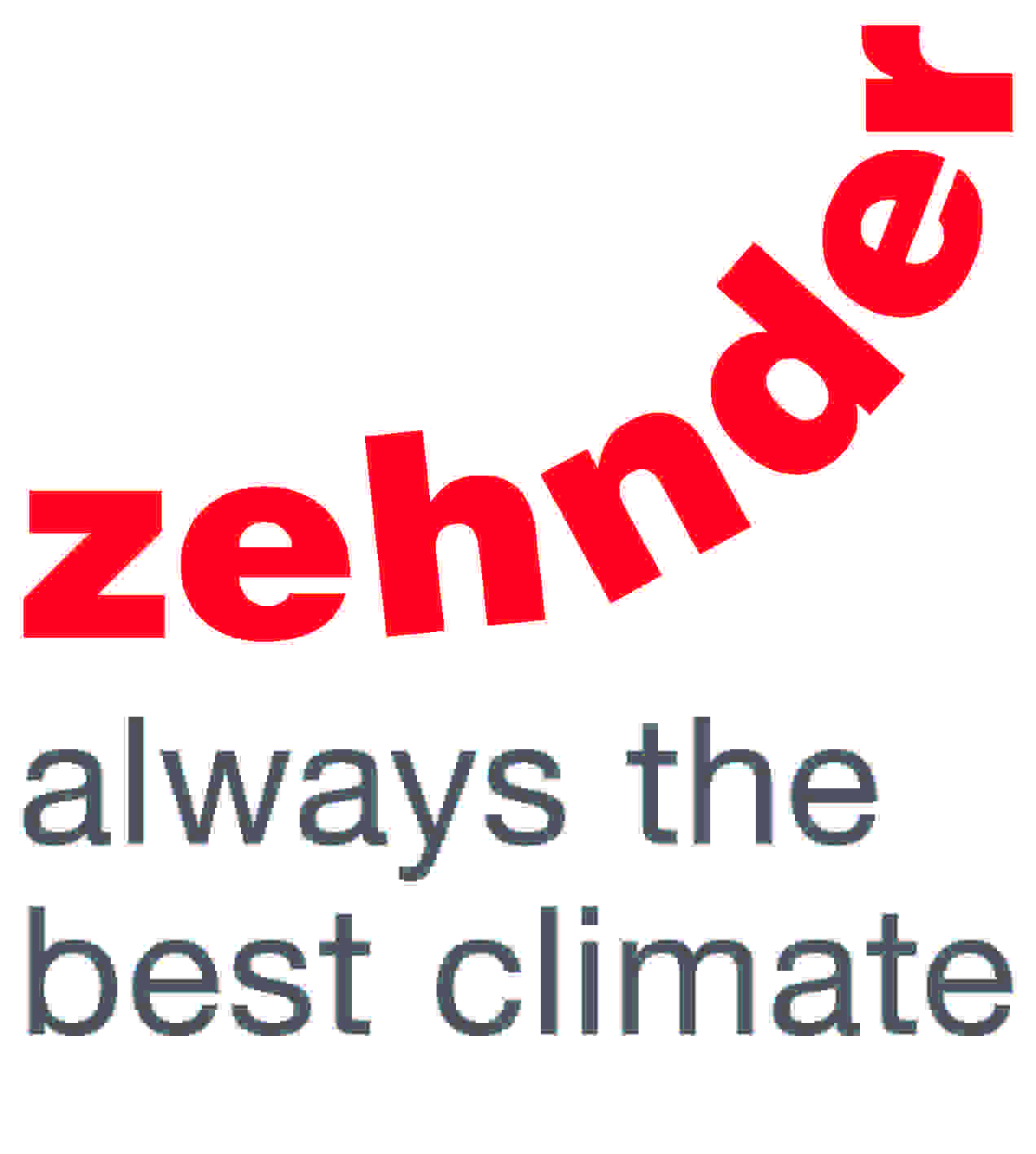
The prime purpose of a ceiling-suspended radiant panel (also known as a ‘foil’ or ‘blade’) is to heat or cool the space to give a comfortable environment for the occupants. Radiant panels are typically used in conjunction with a separate system, such as a dedicated outdoor air system, that supplies the ventilation air and controls the space humidity. The radiant panels, as shown in Figure 1, are supplied with cool or warm water to offset part or all of the room loads. As with other part-centralised air-based systems, this reduces the distribution energy and space requirements compared with an all-air system, and provides opportunities for local control.
As discussed in the May 2018 CIBSE Journal CPD, particularly in respect of radiant systems, the standard measure of comfort, the operative temperature, θc (°C), is calculated1 from
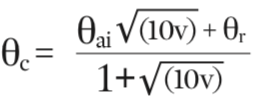
where θai is the air dry-bulb temperature (°C), θr is the mean radiant temperature (°C) and v is the mean air speed (m·s-1) and, if the air speed is taken as being of a similar magnitude to that of natural convection (≈0.1m·s-1), the operative temperature is typically simplified to θc = 0.5·θai + 0.5·θr.
The mean radiant temperature – which is directly influenced by the temperature of a radiant panel (and by every other surface exposed to the occupant) – will, in terms of the operative temperature, have equal impact on comfort to that of the temperature of the air that surrounds the person. The human body aggregates the impact of individual point radiant temperatures, together with all the other parameters that affect comfort. It is this aggregated mean radiant temperature that is combined with (the average) dry-bulb temperature to determine operative temperature.
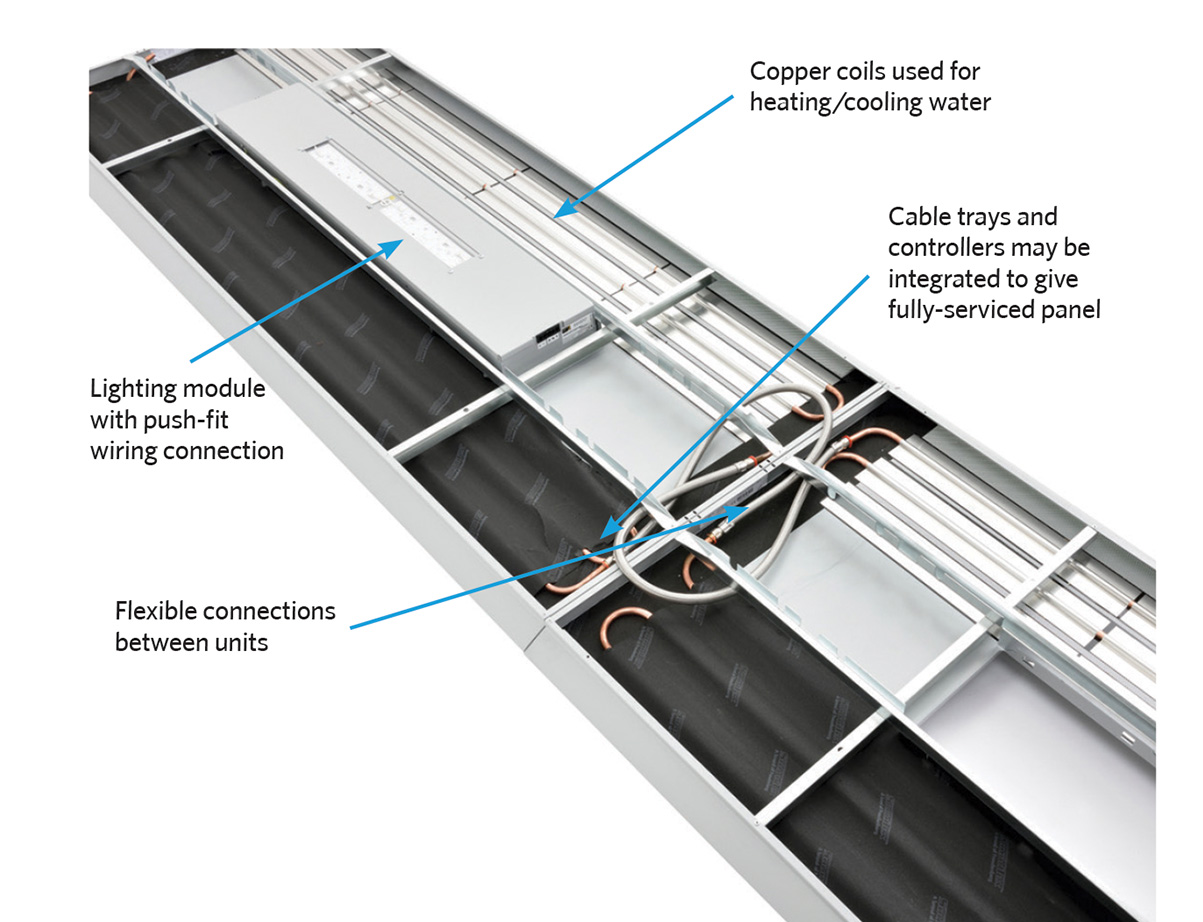
Figure 1: The connectivity of the services in a suspended multiservice radiant panel (showing upper surface of panel with sound absorbent and thermal insulation removed) (Source: Zehnder)
The complex shape and surface types of the clothed human body, as well as the different radiant characteristics (and shape factors) of the surrounding surfaces, make the prediction of comfort particularly challenging, as illustrated in Figure 2. Both CIBSE1 and ASHRAE2 illustrate methods to determine the impact of radiant heating on the occupant comfort by evaluating the view (or angle) factor for radiant heat transfer. However, readily understandable worked examples are best seen in Annex B of BS EN ISO 7726:2001.3
As discussed in a paper by Manabe,4 the position of the person in the room – as well as the influence of other people – will significantly affect the relative radiant shape of the human body. Although the paper reports an evaluation method, it is based on six simple planar, isothermal surrounding surfaces that are unlikely to properly represent an application such as an office or a teaching space, as illustrated in Figure 2.
In a modern, well-constructed building, the internal surfaces and furnishings are likely to be at a similar temperature to that of the air. The surfaces that are likely to deviate most from the mean air temperature are the external surfaces and those of the heating and cooling devices. The value of θr will alter with the position of the occupant and with the surface temperatures.
From experience of installations, manufacturers are able to give guidance on the practical impact in a room or, if the specific information is available, various scenarios may be assessed with dynamic thermal models.
The asymmetry of radiant temperatures will also affect comfort, regardless of the dry resultant temperature. The guidance that is provided by CIBSE, ASHRAE and ISO 77305 is shown in Figure 4. For class 2 (or category B) buildings, ISO 7730 limits the radiant asymmetry for warm ceilings to 7K.
Recently, Safizadeh6 examined the impact of different radiant uniform ceiling temperatures on comfort for a ceiling height of 3m. This was a laboratory-based experiment, and so restricted, necessarily, in its ability to simulate real applications. However, it was particularly interesting in that – unlike the work that underpins much of the guidance of radiant temperatures for comfort – it included not only variations in the ceiling surface temperature, but also the impact of temperature and distance of other surrounding surfaces.
One of the surprising findings – and contrary to expectations – was that the test subjects considered that their head was the most comfortable, even though it was the closest part of the body to the warm ceiling. Their results indicate that, if the radiant ceiling operates at low average temperatures (below or equal to 38°C across the whole ceiling), they were able to provide conditions of neutrality – neither too warm, nor too cold.
In the discussion of the results, they considered that the predicted mean vote (PMV) index does not assess the perceived thermal feeling of the occupants exposed to asymmetric radiation correctly. Specifically, they note that, where occupants are dressed in winter clothing, a radiant temperature asymmetry of 7.5K will still provide neutral conditions.
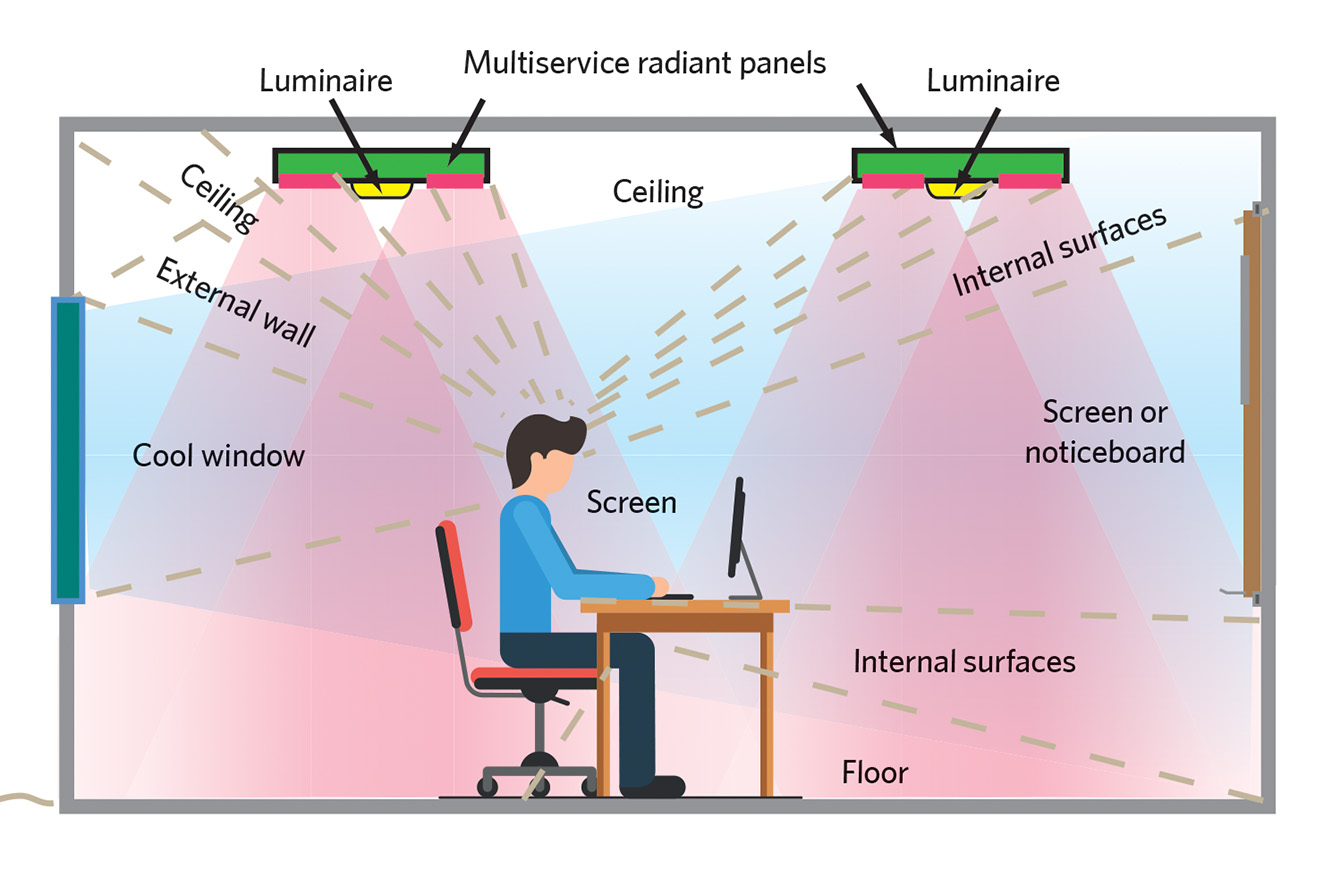
Figure 2: This illustrates the complexity of determining the impact of radiant heat transfer for the evaluation of mean radiant temperature when heating an area such as a seminar room – shown as a much-simplified section that, in reality, is influenced in three dimensions
As suggested by the sketch in Figure 2, when applying radiant panels – as opposed to a whole planar heated ceiling – the panel temperature will have a reduced influence on the aggregated radiant temperature on the top of occupants’ heads. In the example installation in Figure 3, depending on room design loads, the radiant panel ‘active area’ could be one or two sides of the panel face (that is, 33% or 66%) of the 2.2m x 0.9m area that, in such an application, would equate to approximately 11% or 22% of the ceiling plane area.
The guidance from the UK Education and Skills Funding Agency7 is that, in an application such as that depicted in Figure 3, an average panel temperature of 50°C would be appropriate for normally able-bodied occupants. This would provide more than 300W heating from each of the illustrated panels.
In spaces where speech is important – such as in an office or school – reverberation times of around 0.6 to 0.8 seconds are typical to ensure clarity and high speech intelligibility. By comparison, direct sound will take in the order of 0.06 seconds to reach the back of a 20m room.
An occupant in a reverberant room will experience a higher sound level than in a more absorbent room, and speech generated in spaces with reverberation times beyond 0.8 seconds can become increasingly difficult to understand. However, if the reverberation time is too short – such as below 0.3 seconds in larger rooms – the aural environment can sound dull, and this will affect the audibility for those at greater distances from the speaker.
The freely downloadable Acoustics of Schools: a design guide8 is an excellent reference for the assessment and design of spaces where speech intelligibility is important. It indicates that, for applications such as classrooms, the provision of high-level suspended panels of acoustically absorbent material can make a useful contribution to limiting the reverberation time.
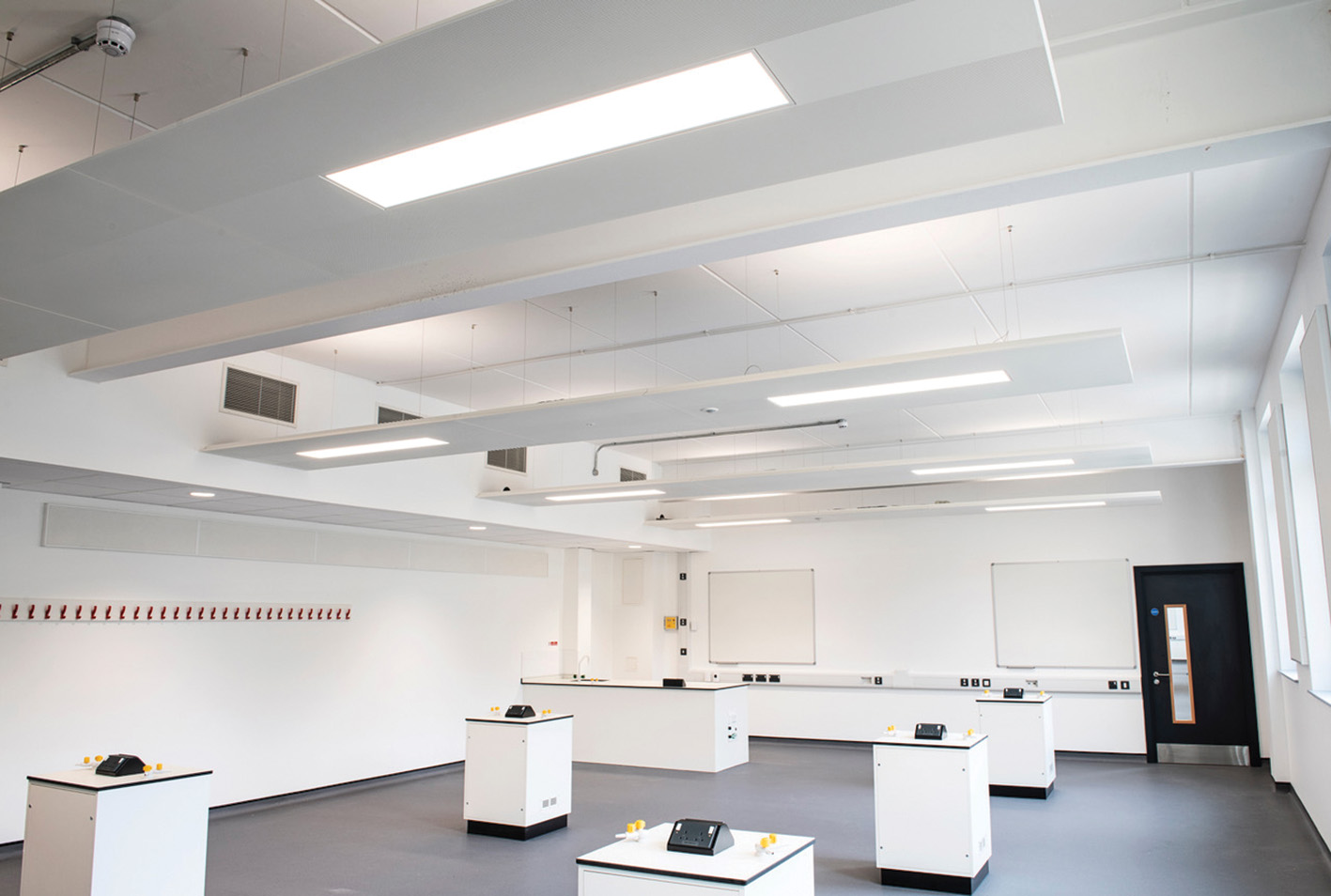
Figure 3:Example application of suspended multiservice radiant panels
The acoustic absorption of multiservice radiant panels is often characterised in terms of equivalent sound absorption area, AR=α·S where α is the absorption coefficient of the absorbent material and S is the area of the absorber. Practically, the complicated geometry of multiservice panels requires that the panel be tested to BS EN ISO 3549, and the equivalent sound absorption area can then be included as part of the Sabine reverberation time calculation, RT= 0.16V/A where V is the room volume and A, the overall room absorption, is the sum of all the products of the room surface areas and their individual sound absorption coefficients, ∑ S∙α.
So, for example, a 2,200 x 900mm multiservice panel suspended 800mm from the soffit, similar to that shown in Figure 5, has an approximate equivalent sound absorption area of 2.1 at 500Hz, based on manufacturer’s test data.10 The same area of plain concrete soffit has a sound absorption area of 0.04. When applied in the Sabine calculation, this will make a significant reduction to the reverberation time in an office or teaching area, so reducing the need for complementary absorbent surfaces.
The lit environment also has a significant impact on comfort and occupant performance. If designed appropriately, it will help prevent fatigue, aid concentration and improve productivity. Beyond the considerations of daylighting, this will be influenced by the position and type of lighting fittings. These will be designed to deliver the required illuminance and distribution of light to the occupied space – including considerations for glare and shading, which are discussed fully in the SLL Lighting Handbook – as well as to ensure appropriate colour rendering and colour temperature.
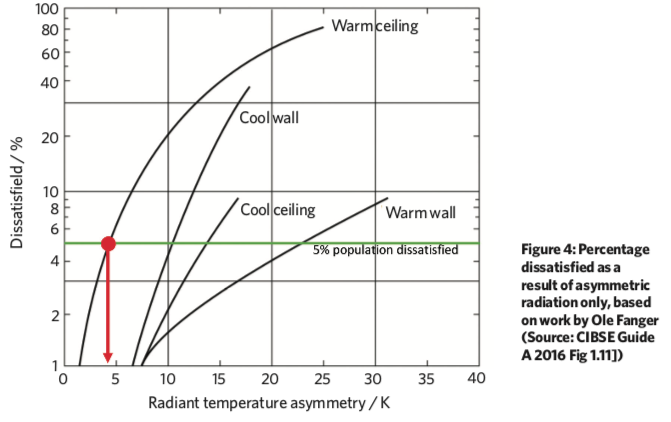
Colour rendering, and the associated colour rendering index (CRI), determine how faithfully colours are replicated under artificial light sources compared with daylight. The CRI compares a light source’s test performance with a reference source – a value of Ra100 indicates a performance similar to daylight, with Ra80 a typically required minimum.
Modern, good-quality LED lamps, which would integrate into a multiservice panel, should be able to meet this requirement of Ra80. However, the simple CRI may not provide the complete story, particularly for LEDs, because even though they typically have poor red colour rendering, this is not exposed in whole-spectrum CRI evaluation. Skin tones, for example, are influenced by the redness of the blood that flows beneath the skin, so a light that lacks red will make a person look pale, or possibly green. Enhanced CRI metrics, such as the so-called ‘CRI R9’ value, may be used to evaluate whether the LED lamp can render red effectively – a CRI R9 value of more than 50 is normally considered good.
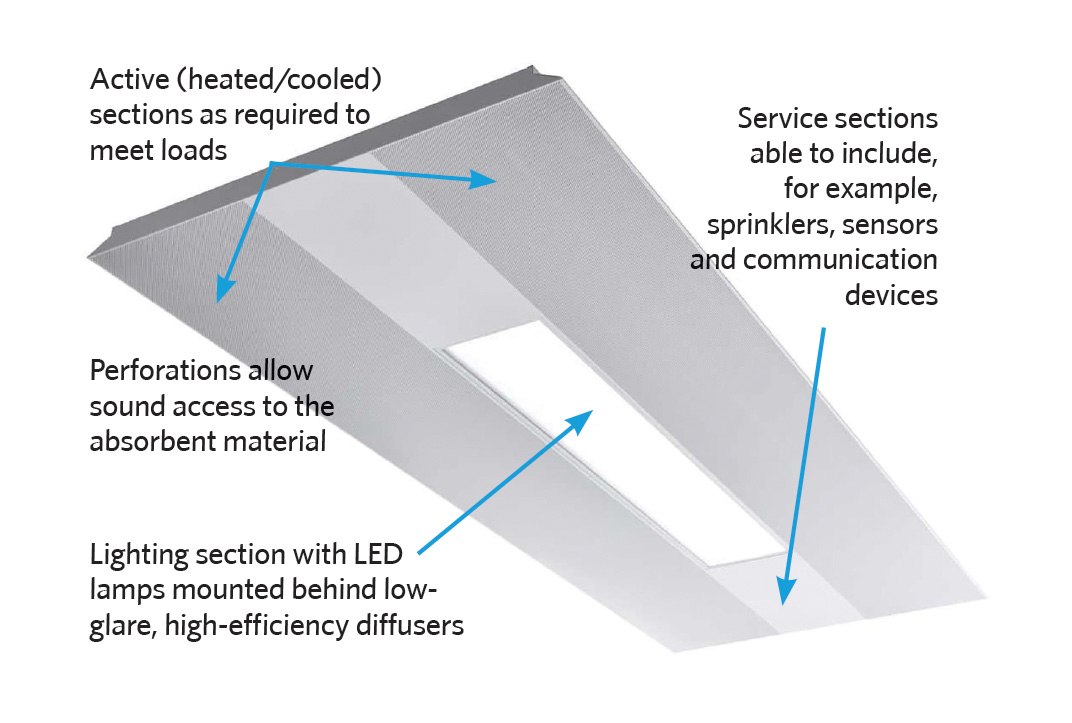
Figure 5: An example of the front face of a 2.2m x 0.9m suspended multiservice radiant panel. The central section is dedicated to the lighting unit, with the option to include additional services in the open areas at either side. A perforation across the panel provides a wipe-clean surface, but allows access to the sound-absorbent material (Source: Zehnder)
As discussed in the December 2018 CIBSE Journal supplement CPD, the modification of colour temperature is increasingly seen as important in maintaining the health and productivity of occupants throughout the working day. With appropriate networked controls and sensors, LEDs integrated into the multiservice panels may be altered to provide, for example, a ‘circadian-friendly’ lighting sequence.
Using individual or group control, and driven by ‘smart’ control devices, wireless mesh networked lighting systems can offer lighting regimes that ensure safety and good practice, but that also allow app-based integration to improve the environment for the individual occupant.
The advent of low-cost mesh wireless controllers – together with more compact and reliable micro-electronic sensors and components working with high-efficiency, controllable LED lamps – have transformed the capability of suspended radiant panels to meet the aspirations of a true multiservice device. With appropriate application, these can contribute to comfortable and effective environments in commercial and educational buildings.
Further reading
CIBSE Guide A – particularly sections 1 for comfort and 5.8, which considers heating plant sizing.
CIBSE Guide B4 – section 4.7 provides information on room acoustics. SLL Lighting Handbook 2018 – this gives detailed explanations of all lighting fundamentals.
References:
1 CIBSE Guide A, Chapter 1, CIBSE 2016.
2 ASHRAE Fundamentals Handbook, Chapter 9, ASHRAE 2017.
3 BS EN ISO 7726:2001 Ergonomics of the thermal environment – instruments for measuring physical quantities.
4 Manabe, M et al, Shape factor calculation and visualisation for the influence of the thermal environment on the human body, 8th Int IBPSA Conference, 2003.
5 BS EN ISO 7730: 2005 Ergonomics of the thermal environment. Analytical determination and interpretation of thermal comfort using calculation of the PMV and PPD indices and local thermal comfort criteria.
6 Safizadeh, MR et al, Experimental evaluation of radiant heating ceiling systems based on thermal comfort criteria, Energies, October 2018.
7 Output specification – Technical Annex 2F: Mechanical services and public health engineering. Version 7, ESFA, 2017.
8 Shield, B et al, Acoustics of schools: a design guide, IoA & ANC 2015.
9 BS EN ISO 354:2003 Acoustics – Measurement of sound absorption in a reverberation room.
10 Foil – integrated lighting, acoustics and services, Whitecroft Lighting – accessed 15 April 2019.
© Tim Dwyer, 2019.