This CPD will consider some key aspects of the thermal environment and their contribution to what is, effectively, the ‘productivity cocktail’.
The focus of this article will be air and mean radiant temperature, air speed, and relative humidity – parameters that may be most readily controlled by the building systems. (CIBSE Journal CPD module 147, in June 2019, drew on a draft of CIBSE TM40 (2020) Health Issues and Wellbeing in Building Services and touched on aspects relating to the visual environment.)
These will combine with other parameters – including the attributes of building users, the space, and the environmental systems – to form the working environment. The recently published edition of TM40 provides an excellent, and accessible, discussion of the broad range of factors that impact the productivity of occupants in buildings.
The productivity of occupants is often key to whether an environment is deemed a success, whether a traditional place of work – such as an office, restaurant, or healthcare, teaching or manufacturing facility – or, possibly, a newly established, ‘distanced’ or isolated workspace.
Productivity may be interpreted in many ways. It can be as simple as being able to watch a film in ‘comfort’; creating a product; or, maybe, to undertake a life-saving medical procedure successfully.
Numerous parameters have been identified as impacting productivity, as discussed by Clements-Croome1, and so – as with the many design challenges in building services engineering – a holistic appraisal of factors is required to maximise opportunity for productivity, while avoiding potential, unintended, deleterious consequences on micro, local and global environments.
As with any evaluation, the methodology used to assess productivity will undoubtedly affect the outcome (see box, ‘Seeking a measure of productivity’). However, as clearly identified2 by Bordass and Leaman, and reflecting their decades of evaluating the performance of buildings: ‘The cat’s cradle of causality and association differs from one building to the next, making it dangerous to be over-assertive about causation without careful appreciation of contexts.’
A popular example of a regularly expressed generalisation that highlights the need for suitable contextualisation is the impact of CO2 in inhaled air on human performance. A typically quoted performance CO2 limit for building occupants is around 1,000ppm, which has been informed by a number of studies, including that undertaken recently by Allen et al,3 as illustrated in Figure 1.
Using a test group of 24 professional-grade employees in New York, USA, over a six-day longitudinal study, this indicates a significant reduction in occupant higher-level cognitive capability as CO2 levels rise towards 1,500ppm.
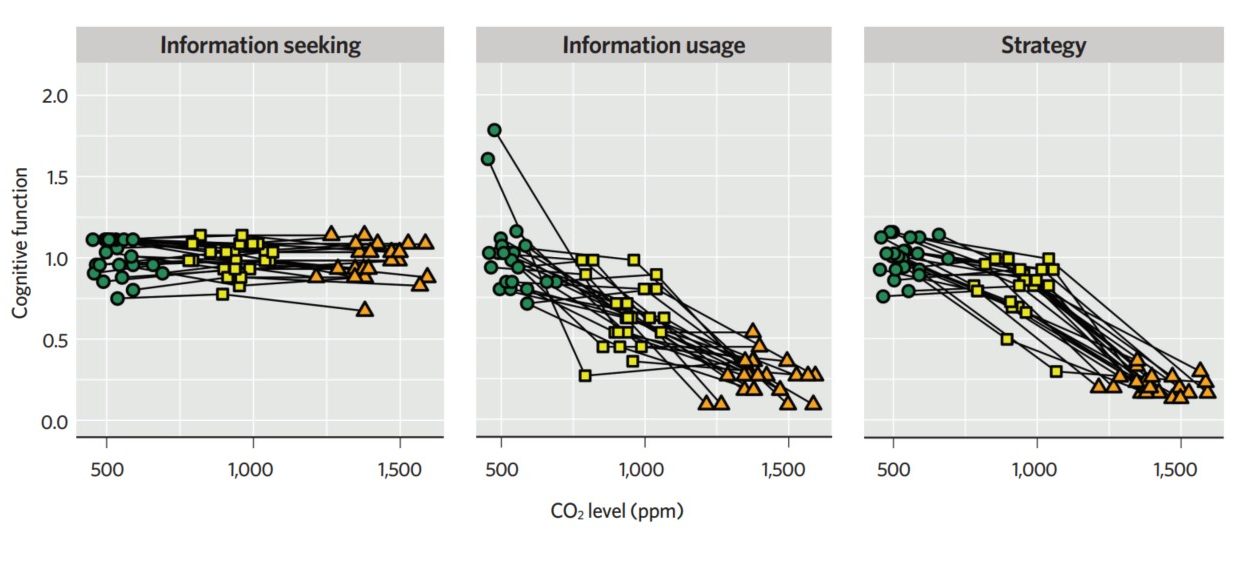
Figure 1: Selected findings from Allen et al summarising SMS experiments undertaken to determine the cognitive performance of occupants in a controlled office environment with different concentration of CO2 (Source: Allen et al3)
In Allen’s experiment, cognitive assessment was performed daily using the Strategic Management Simulation (SMS) software tool. The same tool was employed by Rodeheffer et al,4 although this experiment used submariners as the test subjects. (The test also differed because the subjects had just a 45-minute acclimatisation period to the CO2 levels, and it was undertaken in a test chamber, rather than an office environment.)
However, the outcomes were completely different. At exposure conditions of 600, 2,500, and 15,000ppm CO2 there were no significant differences for any of the nine SMS measures of decision-making for the submariners.
This provides a persuasive reminder that context, the specific occupant population, acclimatisation and adaptation are key determinants in assessing the environment in terms of productivity.
As reported by TM40, there is a consensus that the physical environment affects general employee satisfaction, which will impact productivity in workplaces.
The dry-bulb temperature (DBT) in a space is likely to be the variable with which occupants most readily relate, as well as having some expectation of what numerical value would deliver personal comfort and so aid their productivity. The influence on ‘temperature’ was explored by Sepännen et al by reviewing5 24 field studies that had investigated the relationship between indoor temperature and occupants’ ability to undertake office tasks. From this work, TM40 provides a simplified chart, as shown in Figure 2.
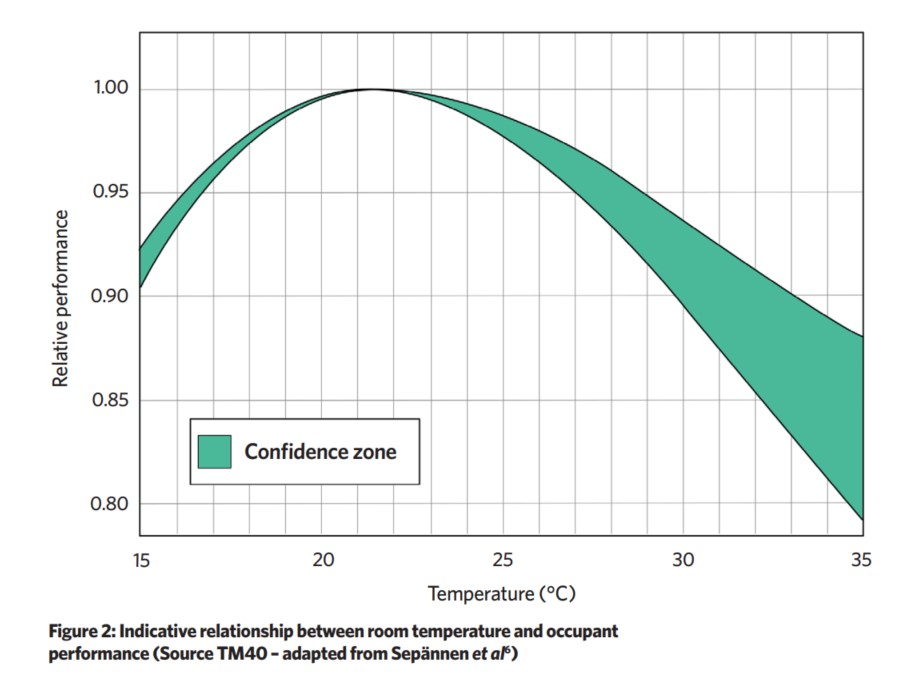
This indicated that the most productive temperature was between 21°C and 23°C (the indoor temperature of this work was not specifically noted as being DBT).
In their influential research, Oseland and Burton6 noted that the impact of temperature was rated among the most important physical indoor environment parameters, and the average effect on task performance was estimated to be 15–20%.
In the case of free-running buildings, optimum temperatures would take account of adaptive approaches that tend to more closely follow the outdoor temperatures.
The dry-bulb air temperature in the occupied zone may be readily monitored using proven technologies. However, it is more challenging to determine the impact of radiant temperature on the individual.
The value of operative temperature – the index of basic environmental parameters that influence thermal comfort – is influenced, at typically low room air velocities, by the mean radiant temperature (MRT) as much as it is by DBT.
As MRT is explicitly related to shape, relative locations and emissivity factors, it is practically difficult to continuously assess the MRT from the viewpoint of individual occupants (although new7 sensing techniques promise future potential).
In spaces with high levels of thermal insulation, multiple-glazed fenestration and solar shading (characteristics of many ‘high-performance buildings’), the difference between the MRT and the DBT may be limited, so the typically convenient approximation of the MRT being close to the value of the DBT may be valid. The challenges in practically assessing MRT has inevitably led to a paucity of measured field studies that consider the impact on the individual’s thermal comfort and productivity. This may be a significant deficiency in the current evidence base, as discussed by Chauduri et al.8
The impact on specific occupants in spaces with asymmetric radiant temperatures and dynamic radiant temperatures is difficult to assess, although recent work undertaken by Barnaby and Pedersen9 has developed a tool that may assist in evaluating this.
The influence of radiant asymmetry can be approximated by employing such techniques as illustrated in section 1.6.6.4 of CIBSE Guide A,10 which engages the work of Ole Fanger to suggest that, in the vertical direction, radiant temperature asymmetry (warm ceiling) should be less than 5K and, in the horizontal direction (cool wall), less than 10K. Similarly, for a cool ceiling the maximum recommended radiant temperature asymmetry is 14K, and for a warm wall 23K.
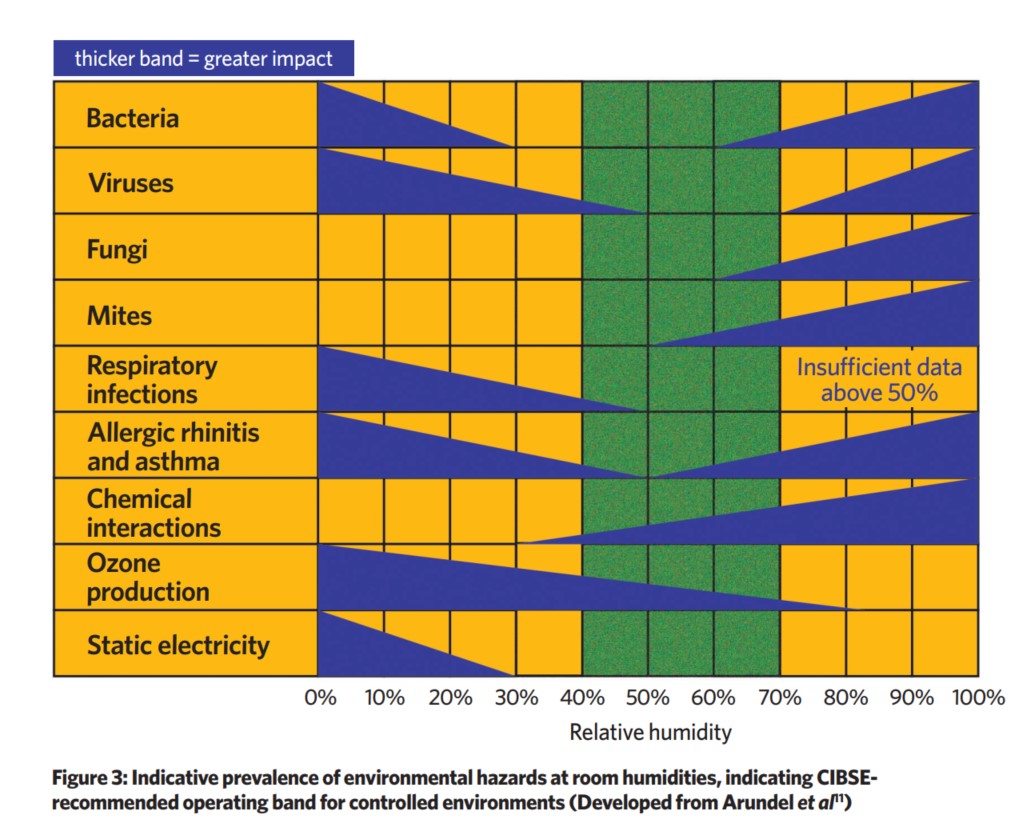
The humidity of the air is likely to noticeably impact thermal comfort at extreme values and, as indicated in Figure 3, the effect on occupant wellbeing is also more pronounced at high and low values of relative humidity.
If the relative humidity is maintained in the comfort range of 40-70%, as recommended by CIBSE,10 this will also provide a reasonable compromise in terms of humidity-associated risks. The adverse impact of higher humidities on comfort may be reduced by localised air movement – for example, as generated by ceiling or desk fans.
Air speeds in an environmentally controlled occupied zone would typically be expected to be between 0.05 and 0.2m.s-1 (and the various comfort criteria are probably founded on this assumption).
Greater air movement will increase the convective and evaporative heat loss from the body, which can provide a useful means of compensating for the discomfort of higher air DBTs.
The ASHRAE chart – shown in Figure 4 – provides this correction in terms of both the temperature rise compared with the base comfort temperature, and the difference between the MRT and the DBT. This chart also illustrates the impact of relative values of MRT and the DBT.
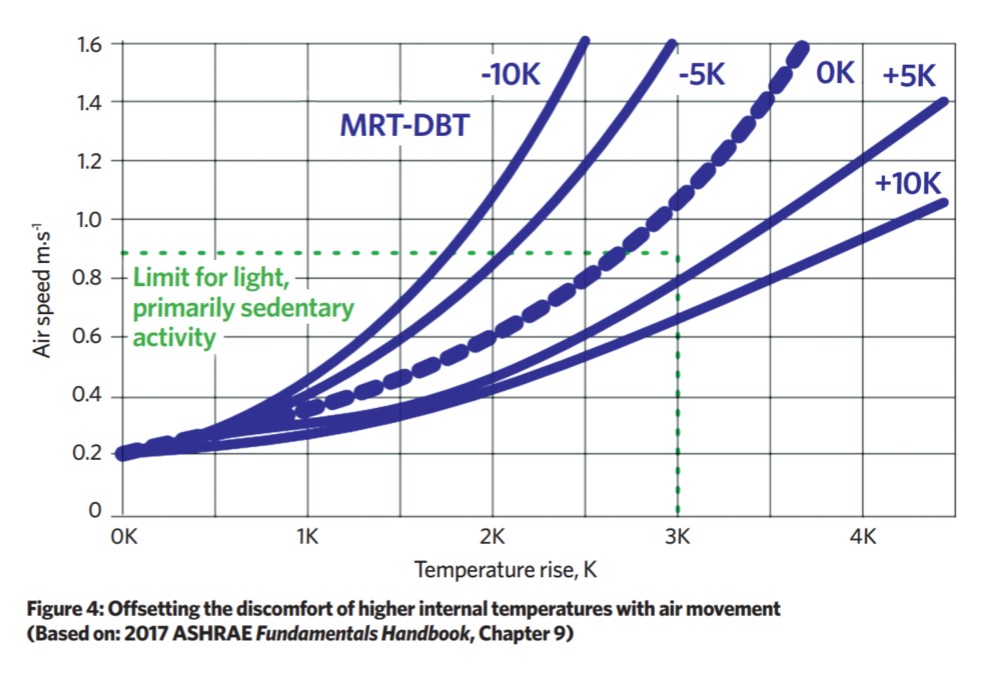
Oseland and Burton report7 that ‘it is generally not acceptable in the UK to include productivity benefits in financial investment appraisal’. As staff are by far the largest cost in offices – typically12 85–90% in the UK and US – a small improvement in staff productivity can result in large improvements in profit margins.
In Seppänen’s review of productivity in offices,13 he notes that even a 1% increase in work performance can offset the annual cost of ventilating a building. In a recent assessment by real-estate firm stok,14 based on aggregating research across the past 15 years, thermal comfort in office environments is potentially influencing productivity by approximately 8%.
As reported in TM40, there is also a consensus from repeated studies and user surveys that providing personal control over an individual’s work environment, along with a rapid-response environment, positively influences satisfaction and productivity.
It is clear that the work of the building services designer and operator can make a significant impact on occupant productivity with appropriate control of basic environmental parameters – but, importantly, this must be appropriately contextualised to deliver a properly balanced and palatable productivity cocktail.
Seeking a measure of productivity
There is no shortage of methods of, and publications on, defining and determining productivity. Quantitative and qualitative methods abound, with some protagonists asserting that quantitative techniques are inappropriate as a means of reflecting – or, possibly, even defining – the complexity and variety of ‘output’, while the qualitative methods are considered, by some, as being unacceptably influenced by bias or preconception.
TM40 identifies four principal methods:
- Self-reported productivity, with the caveat that, although a useful indicator of an individual’s perception, it may overestimate the impact after a change in their environment or, conversely, they may not be able to recognise the influence on their activity.
- Objectively assessed productivity that employs task measurements and standardised tests may struggle to assess creative outputs, with the majority of published materials focusing on applications where tasks and output may be readily measured. However,
TM40 notes that this may overestimate the impact of the physical environment.
- Behavioural analytics is the rising star of techniques as a result of the practical ubiquity of personal smart devices combining with information from sophisticated building monitoring systems.
This provides a highly-accessible method of assessing occupant activity, but may be constrained by privacy concerns.
- Organisation performance, such as output, profitability or personnel data, has the benefit of taking a broader range of factors into account, rather than extrapolating from task-performance results. However, such high-level measures may be difficult to relate to specific attributes of the physical environment
© Tim Dwyer, 2020.
References:
1 Clements-Croome, D J, Creating the productive workplace, 3rd Ed, Chapters 1&2, Routledge, 2017.
2 Leaman, A and Bordass, B, Productivity in buildings: the killer variables: twenty years on, 2016 www.usablebuildings.co.uk/ – accessed 8 May 2020.
3 Allen et al, Associations of cognitive function scores with carbon dioxide, ventilation, and volatile organic compound exposures in office workers: a controlled exposure study of green and conventional office environments, Environmental Health Perspectives, Vol 124-6, June 2016
4 Rodeheffer, CD et al, Acute exposure to low-to-moderate carbon dioxide levels and submariner decision-making, Aerospace Medicine and Human Performance 2018; 89(6):520–525
5 Seppänen, O et al, Room temperature and productivity in office work, LBNL-60952, Helsinki University of Technology/LBNL, 2006
6 Oseland, N and Burton, A, Quantifying the impact of environmental conditions on worker performance for inputting to a business case to justify enhanced workplace design features, Journal of Building Survey, Appraisal & Valuation Volume 1 Number 2, 2012
7 Hongshan, G et al, Mapping comfort with the SMART (spherical motion average radiant temperature) sensor, Building Simulation 2017
8 Chaudhuri, T et al, On assuming mean radiant temperature equal to air temperature during PMV-based thermal comfort study in air-conditioned buildings, The 42nd Annual Conference of IEEE Industrial Electronics Society, IECON 2016
9 Barnaby, C and Pedersen, C, ASHRAE Research Project (RP) 1383, Develop a radiant system module for the simulation and analysis of spaces and systems
10 CIBSE Guide A Environmental design, CIBSE 2016
11 Arundel, A et al, Indirect health effects of relative humidity in indoor environments, Environmental Health Perspectives Vol 65, pp.351-361, 1986
12 CIBSE TM40 Health issues and wellbeing in building services, CIBSE 2020
13 Air, Swegon Air Academy, 2008
14 Attema, JE et al, The financial case for high performance buildings, San Francisco:stok, 2018