As discussed in a UK Parliament briefing paper,1 air pollution is causally linked to an increased risk of several serious health conditions, including heart disease, stroke, certain cancers, dementia, cognitive decline, impaired lung growth, and various respiratory illnesses. The impact of typical ambient (external) pollutants are represented in Figure 1, as summarised by the European Environment Agency (EEA).2
Additionally, volatile organic compounds (VOCs) – such as formaldehyde – can have a range of health effects, depending on the specific VOC concentration and duration of exposure. These include eye, nose, and throat irritation, headaches, dizziness, and fatigue. Prolonged exposure to VOCs can damage the liver, kidneys and central nervous system, and certain VOCs are known – or suspected – carcinogens.
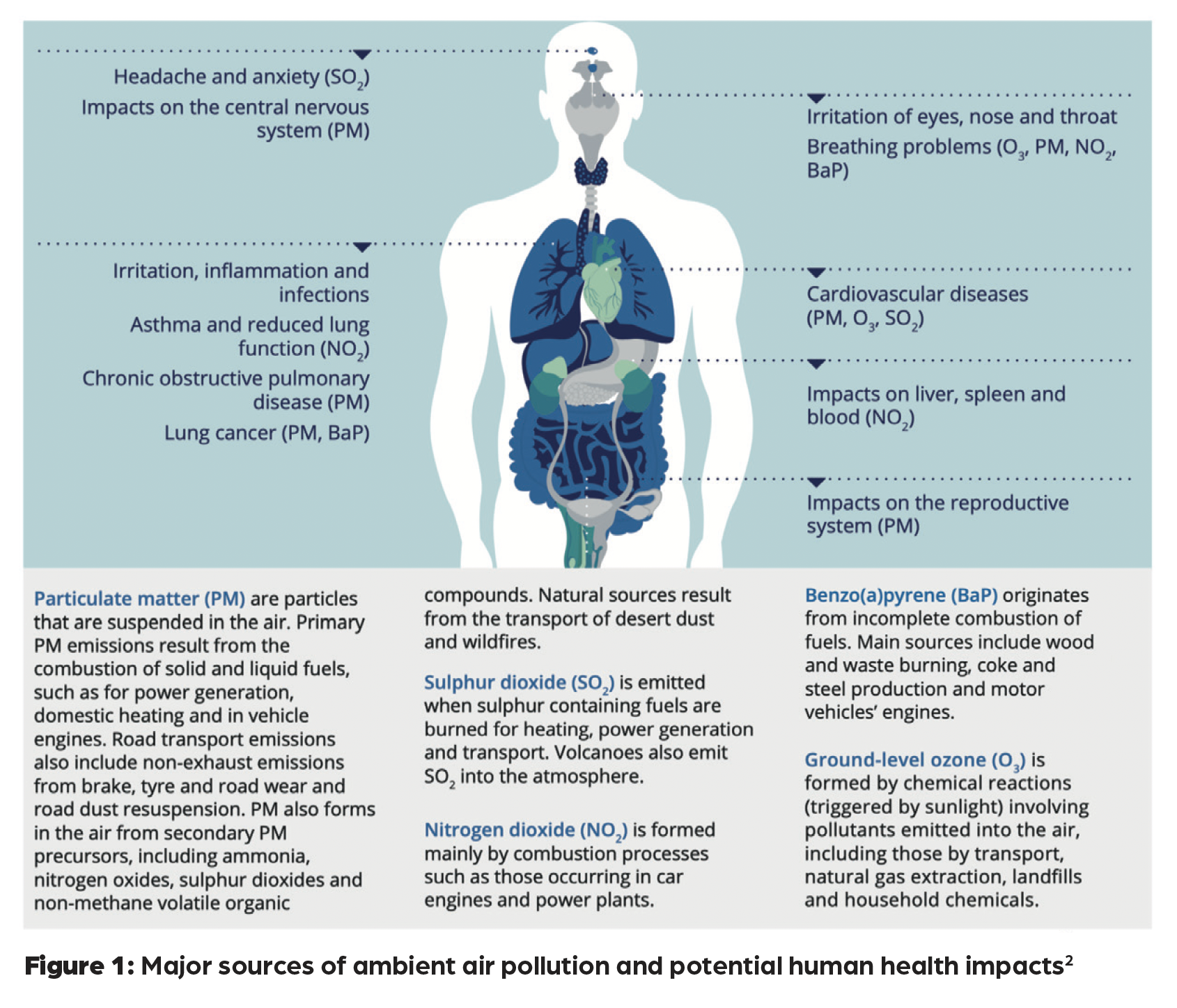
This covers exposure to the headline VOC groups phthalates3 (commonly used to add flexibility and durability to plastics, such as flooring), and per/poly-fluorinated substances (PFAS)4 that are widely used in various building materials, flooring and fabrics owing to their desirable properties like water-, stain- and heat-resistance.
There have been many attempts to quantify the influence of the various sources of pollutants into the internal space; however, individuals are exposed to a wide range of pollutants as they move through different indoor environments, each with its unique set of pollutant sources.
Climate change is likely to adversely impact IAQ by increasing the infiltration of outdoor pollutants including ozone and particulate matter. Higher temperatures and humidity levels promote the growth of mould, dust mites and allergens indoors, contributing to respiratory issues. Inappropriately filtered or controlled HVAC systems can inadvertently circulate these pollutants, further deteriorating air quality.
The recent study5 by Saraga et al. examined 127 international peer-reviewed studies, revealing a significant variation in contaminants affecting IAQ in occupied environments, with no clear or consistent pattern emerging. However, the review identified that off-gassing from building materials, surface coatings and wood-based products dominated as sources of VOCs.
There is no practical method of continuously sensing viruses and bacteria in buildings and HVAC systems so the level of risk will typically need to be assessed and proactive control mechanisms employed as deemed necessary, such as high-efficiency filters and ultraviolet germicidal irradiation (UVGI), and by controlling the volumes of ventilation air.
A 2022 report by the UK Department for Environment Food and Rural Affairs (Defra) Air Quality Expert Group6 highlighted specific IAQ concerns across different environments. In care homes, limited ventilation resulting from restricted window openings can lead to the accumulation of pollutants.
Several research projects have identified inadequate – or improperly controlled – ventilation as a cause of poor IAQ in social housing. As shown by McGill et al.,7 this is not confined to ‘legacy’ homes. They investigated homes built to high sustainable standards, and their findings suggest inadequate IAQ and thermal comfort in the dwellings.
Nurseries and schools, where children spend significant time, often have limited ventilation to reduce heating costs, resulting in high levels of pollutants including carbon monoxide (CO), carbon dioxide (CO2), particulate matter (PM), and VOCs, especially in urban or high-traffic areas.6 Hospitals typically demand specialised consideration of ventilation.8
Some workplaces can present specific air quality risks, particularly in sectors like manufacturing and construction, where exposure to substances such as asbestos, particulates and VOCs is common. As noted by the US Environmental Protection Agency (EPA),9 office occupants may be exposed to a mix of contaminants from indoor pollution sources that are potentially exacerbated as a result of poorly designed, maintained or operated ventilation systems, and unanticipated or inadequately planned building use.
Office surveys undertaken by the EPA identified asbestos and organics from building materials; formaldehyde from pressed wood products; off-gassing from carpets and other office furnishings; chemicals released from cleaning materials, air fresheners, paints and adhesives; ozone from copying machines; biological contaminants from dirty ventilation systems and water-damaged walls, ceilings and carpets; and pesticides from pest management practices.
Laurent et al considered10 both the impact of CO2 and PM2.5 (particles with a diameter of 2.5µm or less) on the performance of office workers and identified the acute impacts on cognitive function associated with poor IAQ, concluding that benefits from reducing
exposures to PM2.5 and CO2 indoors may positively impact productivity, educational attainment, safety and activities where cognitive performance is important.
Chemical reactions, such as those involving nitrogen oxides (NOx) and VOCs in the presence of sunlight and other atmospheric conditions, can form fine particulate matter, known as secondary PM that can contribute significantly to the total PM2.5 and PM10 levels. The UK Health and Safety Executive (HSE) guideline suggests that total VOC levels should not exceed 300µg.m-3 averaged over eight hours, and the formaldehyde recommended limit is 100µg.m-3 over 30 minutes.
The recently published review11 of air-pollution sensors refers to the growing trend of low-cost sensors (LCS) that, as technology develops, allows the wider deployment of sensing devices, which are sufficiently reliable and robust while also being less costly than research- or reference-grade sensors.
The most prevalent PM sensor is optical, which employs LED light scattering to detect particles and is considered as being reasonably accurate for indoor use, but can be affected by humidity and temperature changes. They are likely sufficient for most consumer (and general HVAC) applications and have an accuracy range of ±10-20% compared with reference-grade instruments.
More expensive, laser-scattering sensors provide better accuracy, especially in controlled environments, and are likely to be accurate to within ±5-10% compared with the reference. Regular maintenance, including cleaning and replacing filters, and regular calibration help ensure sensors remain accurate over time.12
Non-dispersive infrared (NDIR) is the most common type of CO2 sensor used in HVAC systems13 (and is the technology specified in the England Building Regulation AD Part F1 for typical commercial spaces). They are favoured for their high accuracy, reliability and long-term stability. NDIR technology relies on the principle of infrared absorption by CO2.
Infrared light is passed through the sensor’s gas chamber and through a CO2 selective optical filter before reaching the detector. The detector measures the intensity of infrared light and converts it into a calibrated electrical signal – higher concentrations of CO2 result in a weaker electrical signal. In ideal conditions, a well-calibrated NDIR sensor can provide readings within ±1-5% of the reference value. CO2 sensors will tend to drift over time, leading to a gradual decrease in the sensor’s ability to accurately measure CO2 levels.
The choice of VOC sensors for HVAC systems often depends on the specific requirements for sensitivity, response time and budget. Metal-oxide semiconductor (MOS) sensors that measure changes in the resistance of a metal-oxide layer when exposed to gases are relatively low cost but are very susceptible to humidity and temperature variations.
The more expensive NDIR sensors can be employed to provide selective measurements of specific VOCs. Similarly-priced electrochemical sensors measure VOCs through a chemical reaction that generates an electrical current, providing high accuracy for a limited range of VOCs over a relatively short lifespan (said to be in the order of three years).
Photoionisation detectors (PID) – at about 10 times the cost of MOS sensors – use ultraviolet light to ionise VOCs, and can detect low levels of a wide range of VOCs (in the ppb range). However, in common with most high-end sensors, these require regular and relatively frequent calibration and maintenance.
Commercial buildings are normally ventilated with outdoor air to replace the vitiated air and to dilute air contaminants created by occupants and their activities (‘anthropogenic’ activity). Standards and guidelines (such as CIBSE Guide A) specify the minimum amount of outdoor air that is to be supplied by ventilation systems based on occupancy conditions (or area-based values for sparsely occupied spaces).
This assumes that the quality of outdoor air is good. If outdoor concentrations of contaminants are continuously or intermittently high, then it is critical to devise control measures to not inadvertently worsen IAQ through ventilation. Office buildings often have transient occupancy, and conditions that are generally below the maximum capacity and, hence, energy may be wasted through over-ventilation.
An approach to solving this problem is demand control ventilation (DCV), which could be provided by the system in Figure 2.
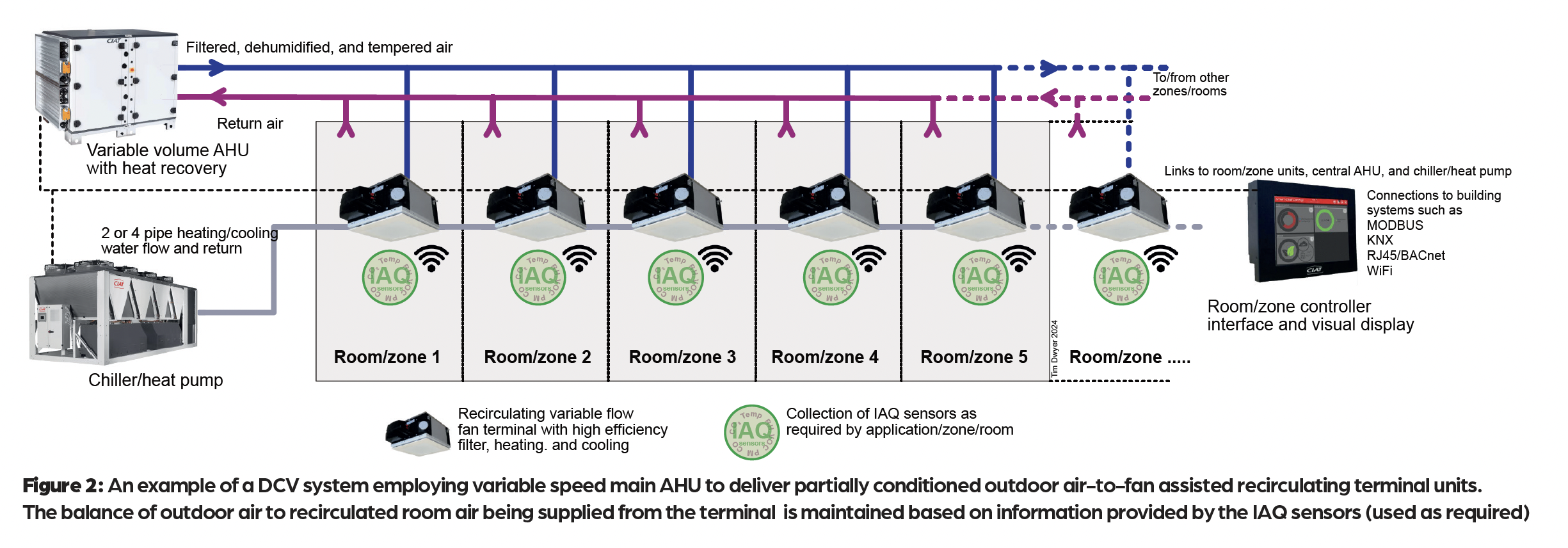
DCV is a building ventilation strategy that adjusts the amount of outdoor air provided to a space based on the occupancy and activity level and, potentially, in response to the levels of specific pollutants in the indoor (and outdoor) air. In a recirculation air system, this could be by modulating the mixing dampers to vary the proportion of outdoor air, or perhaps by simply using variable speed fans and possibly employing volume control dampers to alter the supply of ventilation air to all – or specific areas of – the building.
Since human respiration produces CO2, it has often provided a useful proxy to indicate occupancy levels and, where IAQ is dominated by occupancy-related emissions, CO2-sensing is well established as a means of controlling effective DCV. Sensors, typically placed in the return air ducts or within the occupied spaces, continuously feedback the concentration of CO2 in the air.
As CO2 levels rise above a pre-determined threshold (indicating increased occupancy), the DCV system responds by supplying more outdoor air to dilute the contaminants in the indoor air. Conversely, when CO2 levels are low (indicating reduced occupancy), the system reduces the ventilation rate.
Such systems can also provide reduced, or no, airflow during unoccupied periods – this can be controlled by a combination of sensors and timed switching. Developments of LCS has opened opportunities for more applications of particulate and specific gas sensors that, working alongside CO2 sensing, can offer a more detailed interpretation of the IAQ in situations that may not be dominated by occupant activity. The DCV control systems use this data to adjust the ventilation rates. DCV systems can significantly reduce energy consumption for heating, cooling and ventilating a building by providing ventilation based on real-time needs. This is particularly important in large buildings or spaces with variable use, such as offices, schools and auditoriums.
As noted in CIBSE Commissioning Code A,14 the system designer has a prime responsibility for the provision of sufficient outside air. The systems should include a suitable degree of filtration not only to make incoming outside air as ‘clean’ as possible, but also to remove detritus from the return air from the building prior to its travel through an air handling unit (AHU). There may also be a need to employ more extensive methods of air cleaning to allow the recirculation of otherwise contaminated air.
Understanding the diverse range of contaminants – including particulate matter, VOCs and biological aerosols – is crucial for developing effective strategies to mitigate their impact on health and wellbeing. DCV can provide a useful approach to managing IAQ by adjusting ventilation rates based on real-time data, thereby enhancing air quality while optimising energy efficiency. However, as the understanding of indoor pollutants and their effects continues to advance, ventilation systems and their sensing and control mechanisms will need to evolve to ensure the wellbeing of occupants while meeting standards of environmental sustainability.
© Tim Dwyer 2024.