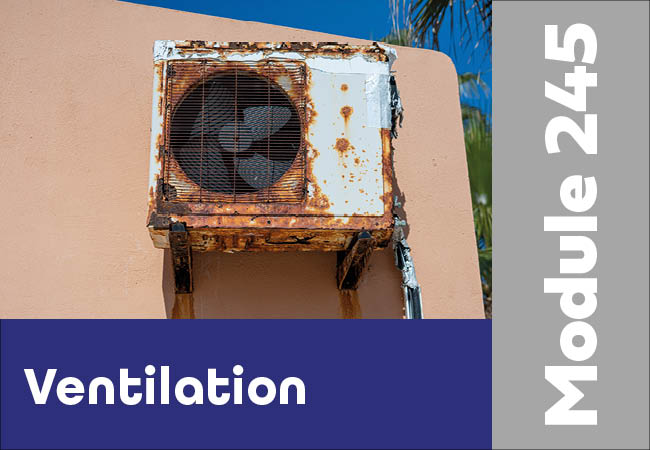
Tropical climates, characterised by high temperatures and humidity, have a significant impact on building services design. The goal is to achieve thermal comfort while protecting the building fabric, reducing energy consumption and minimising environmental impact. This CPD will explore considerations for designing buildings and systems that effectively support the creation and maintenance of a suitable internal environment.
Tropical climates exhibit significant regional variations. Figure 1 illustrates examples of the Köppen-Geiger climate classification that divides the world into zones based on temperature, precipitation and seasonality.
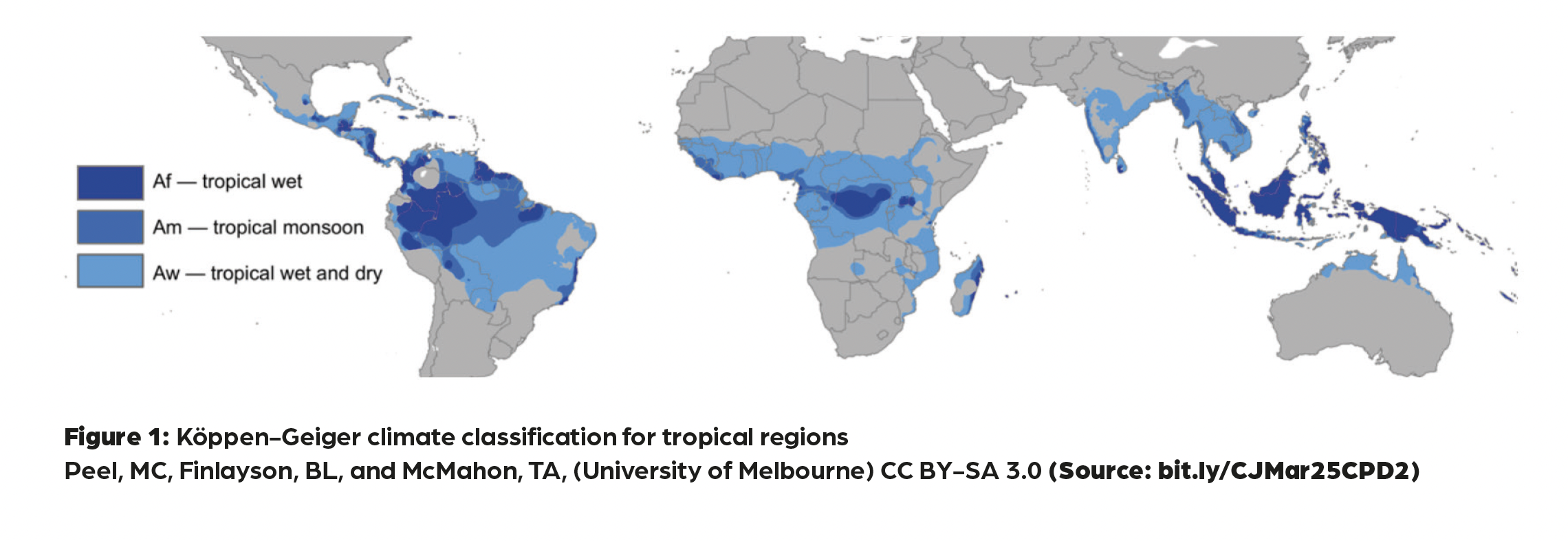
The tropical zones are generally located within 5°-10° latitude of the equator, although in some eastern coastal areas they may extend to as much as 25° north or south. However, tropical climates are not uniform, being affected by elevation, ground cover, larger-scale physiographic features, and the heat island effect.
Delivering and maintaining effective buildings and systems in tropical climates is a significant challenge. When inadequately addressed by systems designers, it can lead to issues such as condensation, mould growth, excessive cooling loads and barely habitable environments. In many areas, designers must consider the possibility of strong winds, heavy rainstorms and flash floods.
As highlighted in guidance1 from the Western Australian Government (WAG), a primary cause of failure is often a lack of understanding of regional conditions by designers, builders and operators who lack experience in tropical environments. There are many regional and tropical-focused codes that are designed to promote the creation of effective buildings for tropical climates.
Directly applying more generic building codes developed for temperate climates to tropical regions can present challenges because of the unique environmental conditions, building practices, infrastructure, and availability of local materials and expertise. People adapted to tropical climates, such as indigenous groups and long-term residents, perceive comfort – especially humidity – differently2 from those who work and live remotely in temperate regions.
Design choices that seem intuitive to a remote designer from a temperate climate may not be suitable for tropical environments. Poorly designed environmental systems reach beyond inadequate thermal performance, encompassing potential building failures, health issues and increased operational costs. Humidity is a key concern, and resulting condensation can lead to mould growth and significant building material damage, such as timber rot, corrosion and reduced insulation effectiveness.
Building design in tropical climates historically3 has been based on passive design principles to maximise natural ventilation, moderate solar gain and exploit natural cooling as a route to delivering occupant comfort. Successful designs account for the specific microclimate of the building’s location, including factors such as the surrounding shading, wind patterns and any local heat island effect. These were traditionally exploited, as in the example of the Malay house illustrated in Figure 2.
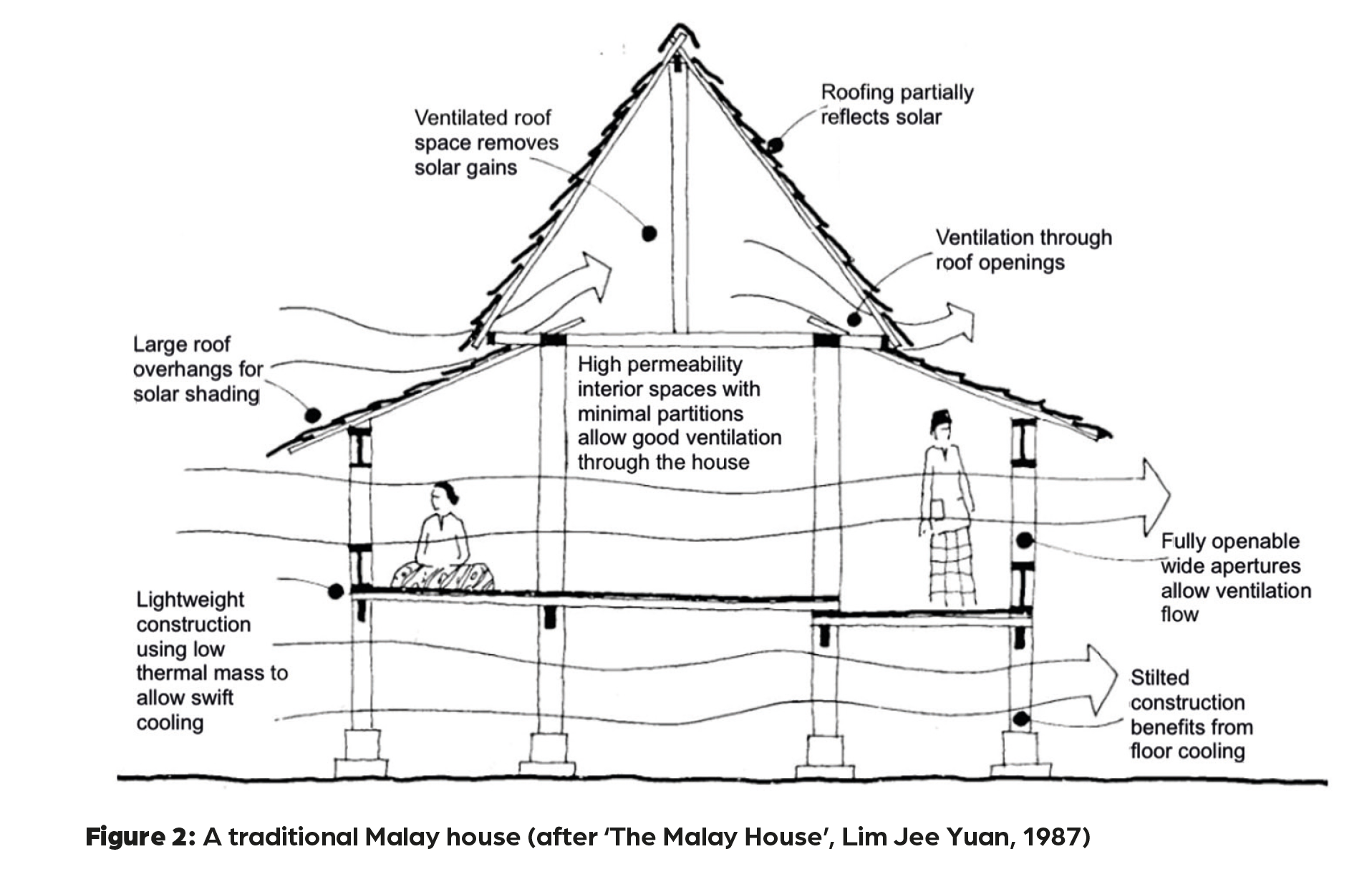
Passive solar techniques, such as orientating buildings towards the sun and selecting materials with favourable thermal mass or light-dispersing properties, can significantly impact the building’s thermal performance. Single-glazed façades can be more energy efficient than double- or triple-glazed systems, if appropriately specified.
Shading can make a significant impact, with horizontal shading more effective than vertical shading because of the high solar altitude. Roofs will benefit from high solar reflectivity to minimise heat gains.
The wind climate can usefully influence the building’s form and orientation, since prevailing winds can assist in ventilating internal and external spaces, as well as increasing heat transfer from the building’s external surfaces. This will guide the choice of building characteristics, such as wide openings in the external envelope, high and low ventilation openings, high ceilings, and wind-catching devices.
The concept of ‘layout permeability’4 relates to the building layout’s impact on the flow of air (as opposed to the building envelope’s ‘fabric permeability’), and this will determine the opportunity for effective wind-driven cross-flow and stack-induced natural ventilation. Openings in the building envelope designed for ventilative purposes need to be well considered so that they can also be suitably protected against encroaching fauna, as well as providing appropriate security and privacy.
Where stack ventilation is employed, it is unlikely to be useful when internal conditions are cooler than the outside, as warm, humid outdoor air will be drawn down into the space – some form of opening control will be required to overcome this. Unfortunately, many tropical locations are not immune to the challenges of poor external air quality, which may limit the application of natural ventilation solutions.
Successful passive design will use climatic conditions to reduce the need for air conditioning – maximising thermal exchange with the environment through natural air movements. Ventilative air movement can be used to aid the removal of internal heat gain, while also improving comfort and personal cooling by increasing evaporation from the body.
Because of this, people in tropical areas tend to prefer slightly elevated air speeds compared with those in temperate zones. A slightly elevated design room temperature with greater air movement is desirable to minimise cooling loads.
Carefully considered selection of building materials and form can minimise heat gains and maximise heat losses. Insulation can provide significant benefits, particularly when installed appropriately in roof structures in both passive and actively controlled buildings.
However – as with any material that offers a significant thermal resistance – when used in buildings that are mechanically cooled, exceptional care needs to be taken to ensure that there is no air leakage, nor vapour transfer through (or alongside) the insulation. Otherwise, there will be a very high risk – and practical certainty – of condensation that, unless able to reevaporate quickly, is likely to adversely affect the building fabric.
Inappropriately located insulation may also reduce the building’s ability to reject heat at night. In areas with minimal diurnal temperature swings, the application of thermal mass may not be desirable if it absorbs and retains too much solar radiation – lightweight buildings that cool quickly in the evening may be preferable.
Dehumidification is a key aspect of the cooling load. Traditional design practice in temperate climates tends to assume a constant relationship between sensible and latent heat loads, which is often inaccurate for tropical applications.
As highlighted in the useful guidance1 produced by WAG, air conditioning systems in humid areas must be primarily designed to manage humidity, as well as control temperature. The dew point of outdoor (ambient) air is frequently above the setpoint temperature of air conditioned spaces, meaning condensation can occur anywhere outdoor air contacts a cooled surface.
WAG notes that setting air conditioning dry-bulb temperatures at, or close to, 27°C can still provide comfort in conjunction with increased air movement from ceiling fans, potentially providing an effective temperature (ET) of 24°C. (Unlike the CIBSE operative temperature, ET additionally incorporates humidity.) A higher dry-bulb temperature may also reduce running costs, as well as the risk of condensation and mould. Thermal buffer zones, controlled at intermediate temperatures, can help reduce thermal shock when moving between indoor and outdoor spaces.
For larger spaces, air conditioning requirements should ideally be considered in zones; such zones may not be immediately apparent and may require parametric computer modelling to establish zones of environmental control within the building. Air conditioning installations, whether centralised or decentralised, should be designed to meet the needs of every zone, and the control regimes related to the use of each zone.
Mechanically cooled buildings should be slightly pressurised by ensuring that the supply air volume flowrate exceeds that of the total exhaust flow, preventing the uncontrolled ingress of warm, humid air. However, as WAG1 notes, effective pressurisation is only achievable in buildings with a fully encapsulating insulated vapour barrier.
Tropical regions are likely to experience consistently high temperatures, intense solar radiation and high humidity levels throughout the year, which places a significant load on cooling systems – the design must, therefore, account for these. Systems primarily focused on sensible cooling may not adequately remove moisture from the air. This can happen when oversized systems quickly reach the set temperature and shut down before dehumidification is complete – keen sizing of cooling equipment may be an advantage in terms of moisture control.
To mitigate this, certain system configurations can be employed. For example, the WAG guidance1 recommends using multi-circuit cooling coils instead of a single continuous coil. This approach maximises the coil surface area below the air dew point, even during periods of reduced sensible loads, improving dehumidification.
Centralised systems may offer advantages in terms of diversity and backup capacity, while decentralised systems can be more flexible. In centralised air conditioning systems and dedicated outdoor air systems (DOAS), incoming air must be conditioned before it enters the space. Air quality in tropical cities may be poor as a result of emissions and low wind speeds, so, in such locations, ventilation systems must address the requirement for outdoor air while also filtering out pollutants.
The dehumidification of fresh air accounts for a large portion of a tropical building’s cooling load, and handling latent and sensible loads separately can provide a more resource-efficient solution. Water vapour may be removed with a variety of techniques, including: rotary dehumidifiers (employing adsorbent such as silica gel); liquid desiccant dehumidifiers (employing liquid absorbents such as lithium chloride); heat pipes; chilled water or direct expansion (DX) coils; and membrane dehumidification (see boxout).
Membrane dehumidification
Membrane-based dehumidification units operate by selectively separating water vapour from the air using a specialised membrane, and can function as standalone units or as pre-dehumidification components within air conditioning systems.
The dehumidification process is driven by the vapour pressure difference between the incoming humid air and the relatively dry exhaust air from a building. The membrane unit typically features an air-to-air cross-flow moisture exchanger, similar in design to the heat exchangers used in energy recovery. Humid primary air and dry secondary air pass on opposite sides. The membranes are often hydrophilic and made from polymeric or nanoporous (micro/meso/macro) materials. Common membranes include hydrophilic materials such as polyvinyl alcohol (PVA) and nanoporous metal-organic frameworks (MOFs), microporous zeolites, and mesoporous silica-based materials. Moisture from the incoming air is adsorbed onto the membrane surface, transported through the membrane, and desorbed into the exhaust air stream. While the dehumidification process itself does not require electricity, additional fan power is necessary to move air through the cross-flow exchanger.
Current technologies achieve maximum vapour transfer efficiencies of approximately 50% under tropical conditions.
These can be arranged to focus primarily on moisture removal without sensibly overcooling the space. Where absorbent and adsorbent processes are used, there may be opportunities to use waste heat or solar thermal sources for the regeneration process. Heat recovery systems, such as thermal wheels, can be used to transfer heat between air streams, but may be less viable in tropical climates.
Environmental systems should be designed for controlled part-load conditions, as they often operate below their maximum capacity. Variable-speed drives and other modulating technologies can enhance system performance and save energy.
However, part-load operation can be troublesome with variable-speed cooling systems, as they need to be carefully selected and controlled to ensure they still remove moisture when humidity is high while sensible cooling loads are low. Rather than a single large unit, it may be advantageous to select multiple staged units that can each work nearer peak capacity.
Conditioned air should be supplied to the space through diffusers that induce room air into the primary air supply, so reducing the risk of condensation by ensuring the cooler supply air is mixed with room air before contacting any building surface.
Displacement ventilation – with relatively large volumes of air supplied at low velocity near the floor with relatively high temperatures – may provide an alternative means of supplying air while reducing the risk of condensation. Suitably regulated demand control ventilation (DCV), using CO2 sensors, can adjust the mechanical (or, potentially, natural) fresh air intake based on occupancy levels, which can reduce the space dehumidification load.
Unitised, decentralised equipment – such as fan coil units (including variable refrigerant flow [VRF] and ‘split’ air conditioners) and radiant cooling – may be used to reduce the load on the mechanically supplied air.
Such systems need careful selection and sizing so that they are suitably controllable to meet coincident sensible cooling and to meet any dehumidification requirements, even at part-load conditions. Any radiant cooling that is used to supplement convective cooling must avoid creating surface temperatures below the room air dew point so as not to produce uncontrolled condensation.
Mixed-mode systems can be employed to combine both passive and active cooling methods to enhance comfort and minimise energy use. For example, natural ventilation may be suitable as free cooling during cooler or unoccupied periods, particularly at night.
The WAG guidance warns that any part of mechanical systems with a surface temperature below approximately 25°C is susceptible to condensation and needs fitting with a suitable insulated vapour barrier. Airtightness of ductwork is essential to avoid leaks of cooled air, which can also pull untreated air into building cavities, leading to condensation and mould growth. Ducted fan coil units should always include a separate insulated safety drip tray below the unit.
Equipment needs to be acclimatised for tropical conditions, especially if installed outdoors. This involves using materials and components that are resistant to corrosion, ultraviolet radiation, and extreme temperatures. Outdoor equipment needs to be protected from corrosion, and should withstand a temperature range of 13°C to 32°C, along with heavy rainfall.
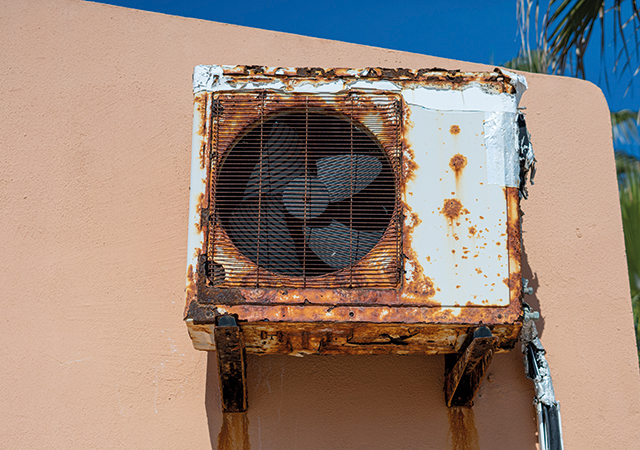
Figure 3: A condensing unit ravaged by the climate (Credit: iStock.com/Migyel Angel Flores)
The alternative is illustrated in the example of Figure 3. Refrigeration plants must be designed to withstand high ambient temperatures and humidity levels. Any externally mounted equipment will – depending on location – be exposed to the local flora and fauna, and will require suitable protection and a regular inspection schedule.
Renewable energy sources are particularly pertinent in tropical regions because of the consistent availability of solar radiation and the need to reduce reliance on fossil fuels for cooling and other energy demands. Solar PV is a key renewable energy technology for tropical climates and because of the high proportion of diffuse light, thin-film PV modules may perform better than crystalline silicon-based cells. PV panels can be integrated into various parts of a building, such as façades and roofs, and can act as sunshades.
The reliability and serviceability of the plant are key considerations. Locally sourced materials and equipment are generally beneficial, as they are better understood by local artisans. For building longevity and beneficial impacts on life-cycle carbon and cost, the ease of maintenance and operation by local teams is paramount.
It is essential to programme in appropriate testing and commissioning to ensure installed systems operate as designed and achieve the desired safety, comfort and efficiency levels. Properly planned and delivered contextualised education of building users and operators plays an important part in the development process to ensure that they benefit from the building’s best performance. l
© Tim Dwyer 2025.
Further reading:
This CPD article provides only a brief overview of delivering effective built environments in tropical climates. Readers are encouraged to explore further to develop a more comprehensive understanding. The following publications were invaluable sources for this article, and I highly recommend them for deeper insight:
- CIBSE, Buildings for extreme environments – Tropical – free to CIBSE members.
- The ASHRAE Guide for Buildings in Hot & Humid Climates – purchase through ASHRAE.
- UN-Habit, Sustainable building design for tropical climates – Principles and Applications for Eastern Africa – free download.
- Government of Western Australia, Northern Regions Heating, Ventilation and Air Conditioning Design Guideline – free download.
References:
1 Northern Regions Heating, Ventilation and Air Conditioning Design Guideline, Government of Western Australia, 2017 – bit.ly/CJMar25CPD1.
2 Vellei, M et al, The influence of relative humidity on adaptive thermal comfort, Building and Environment 124 2017.
3 Tropical-humid architecture in natural ventilation efficient point of view – A Reference of Traditional Architecture in Indonesia.
4 Sustainable building design for tropical climates – Principles and Applications for Eastern Africa, UN-Habitat, 2014.