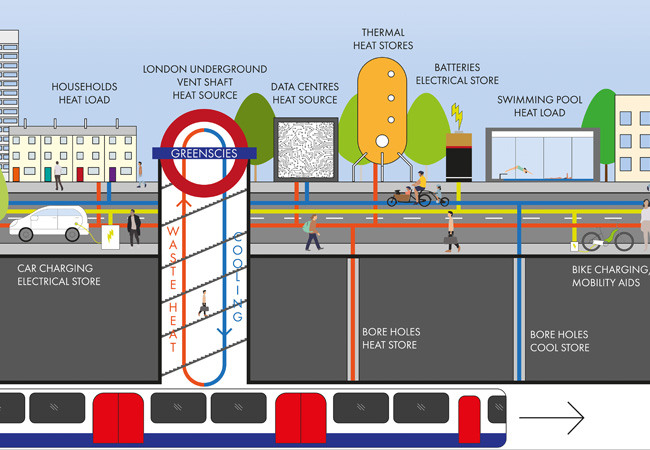
The increasing proportion of Grid electricity generated from renewables is continuing to drive down its carbon intensity – which is good news for the environment. The downside, however, is that sources of renewable energy – such as wind and solar – are intermittent; the more the Grid is dependent on renewables, the more likely it is that there will be large variations in the amount of electricity available.
The situation will be made worse by the progressive move to electric vehicles and the increasing use of heat pumps to provide heating and cooling for buildings, as well as the potential increase in peak loads on the network, making it even more difficult to balance generation with demand.
In Islington, London, the Green Smart Community Integrated Energy System (GreenSCIES) has been launched as one of a tranche of projects assessing the feasibility of using local energy systems in towns and cities to help balance grid demand, improve sustainability and tackle fuel poverty. In Oxford, Energy Superhub – a project using giant batteries– is under construction, with a similar aim.
GreenSCIES, Islington
GreenSCIES has just started its two-year design phase. When complete, it is projected to deliver low carbon heat and power to an estimated 10,000 Islington residents in 3,500 homes, and up to 70 local businesses. It is predicted the scheme will save an estimated 80% of carbon emissions compared with a conventional gas-fired boiler-based system.
The project is a partnership between London South Bank University (LSBU), Islington Council, Transport for London, and a consortium of small and medium-sized enterprises. It is one of 10 local smart energy pilot projects that were awarded a proportion of £21m funding by UK Research and Innovation (UKRI) earlier this year.
The starting point was an initial feasibility study by LSBU, which looked at the heating and cooling demand from existing buildings in Islington. Fundamental to the GreenSCIES project is an ambient heat loop, which enables the exchange of heating and cooling between buildings on the network to deliver extremely efficient, low carbon heating and cooling while ensuring the system remains in balance thermally.
To help in this task, the system includes hot and cold aquifer thermal storage. ‘We did some modelling in Islington based on using an ambient loop to exchange heat between different applications,’ says Graeme Maidment, professor of heating and cooling in the School of Engineering at LSBU, and director of GreenSCIES.
Buildings connected to the system will use a heat pump to extract heat from the heat loop or to reject heat into it when operating in cooling mode. The system is an iteration of the building energy network currently in place at LSBU (see ‘Intranets for heat’, CIBSE Journal, May 2019). A major advantage of using an ambient temperature heat loop is the opportunity it presents to capture waste heat from local sources.
AI will help flex electricity demands from heat pumps and EV charging in response to price signals from the Grid, battery storage, and the intermittent output of solar power
GreenSCIES is proposing to use a 2km ambient pipe loop to capture waste heat from secondary heat sources, including two local data centres and London Underground’s York Road ventilation shaft. In addition, the heat loop will link all 16 of the project’s mini-energy centres. The scheme will also connect to solar photovoltaics (PVs) installations, large-scale battery storage, electric vehicle charging points and, of course, to the electricity Grid.
The decentralised energy centres are effectively a ‘microgrid’, flexing heat pumps, PVs, large-scale battery storage and electric vehicle batteries to enable the system to respond to electricity Grid demand and tariffs. Artificial intelligence will be used to help flex electricity demands from heat pumps and electric vehicle charging in response to price signals from the Grid, battery storage and the intermittent output of solar power.
‘By the end of two years, we’re aiming to have a shovel-ready design available,’ says Dr Catarina Marques, senior research fellow at LSBU. The team wants the project to be tendered and for an energy service company (Esco) to construct and run the system.
The impact of LSBU’s research for GreenSCIES will reach beyond the capital, and will be applicable whenever there are sources of unwanted heat available. In the West Midlands, the project team is investigating the use of waste industrial heat and energy stored in canal water. It is also looking at using flooded, disused mines in Sheffield, in a similar way to that of the Mijnwater (Minewater) project in Heerlen, the Netherlands, which is based around two flooded mineshafts, one kept warm and the other cold to provide seasonal storage.
Energy Superhub Oxford
In Oxford, another UKRI-funded demonstration project is trialling a combination of battery storage and ground source heat pumps (GSHPs) to store energy and help balance the Grid.
The Energy Superhub Oxford is a three-year, £41m scheme based on large-scale battery storage. This demonstrator project includes rapid vehicle charging, hybrid battery-energy storage (a combination of lithium-ion and vanadium flow batteries), low carbon heating and smart energy management, and is anticipated to go live by the end of 2020.
It is being led by battery-storage company Pivot Power, which has already placed an order for the batteries for this project. These are big; the lithium-ion battery is 50MW, housed in 19 shipping containers, and will be linked to a National Grid electricity substation in Oxford. The battery will store energy from the Grid when price and demand are low and supply is high; it will release energy back to the Grid when price and demand are high and supply is low. There is also a 2MW vanadium flow battery – otherwise known as a flow machine – manufactured by RedT Energy (see panel ‘What is a vanadium flow machine?’). Unlike lithium-ion, its electrolyte does not degrade with use, so the project will investigate how to integrate the technologies to reduce degradation in the lithium-ion battery.
The scheme also includes electric vehicle charging on a private wire network, to give 25MW of rapid charging for 100 cars, while – on the heat side – the project is installing shared ground arrays for 300 properties, each with its own GSHP. Smart software will manage the energy storage, electric vehicle charging and heat pumps, to help balance the Grid.
The heat pumps, manufactured by Kensa, will be controlled using Switchee smart controls linked to a cloud-based platform that combines property information with time-of-use tariffs to produce an optimised heating schedule.
The Kensa heat optimisation platform will use the fabric of the property to store heat energy ahead of peak energy times by raising the temperature of the building when electricity is cheap, to allow the GSHP to turn off when the cost of electricity is most expensive.
As electricity will be cheaper when wind and solar generation are high, load shifting heat will help reduce carbon emissions. The system is currently under construction and set to go live at the end of the year.
What is a vanadium flow machine?
The RedT energy-storage machines employ vanadium ions, in different oxidation states, to store chemical potential energy.
A conventional battery stores chemical energy within an electrolyte solution; a vanadium flow machine contains two different electrolyte solutions, each in a separate tank. When the vanadium flow machine is charged, one electrolyte is positively charged and the other negatively charged. For the battery to provide power, the electrolytes flow through a fuel cell stack on opposite sides of a proton exchange membrane.
Their opposite charges create a gradient that drives an external current. There are two main characteristics, unique to vanadium flow machines, that make them suited to utility-scale storage. First, unlike conventional batteries, power output is independent of energy-storage capacity.
Output depends on the size of the membrane stack (the engine), while the energy storage capacity depends on the size of the electrolyte tanks. Neither constrains the other, although the ratio of storage to power determines how long the batteries can run without recharging. Power can flow undiminished as long as there is fresh electrolyte to circulate through the stack.